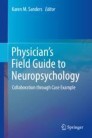
Physician's Field Guide to Neuropsychology pp 435–456 Cite as
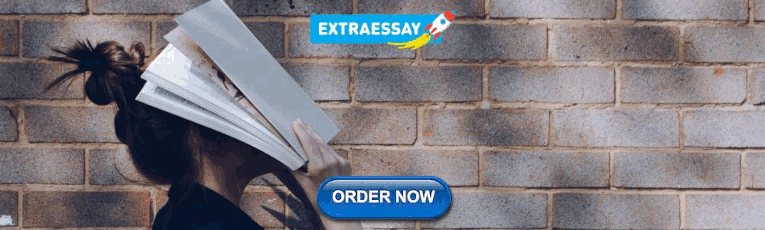
Traumatic Brain Injury Rehabilitation Case Study
- Samantha L. Backhaus 2 &
- Ana Durand-Sanchez 3
- First Online: 23 May 2019
1016 Accesses
1 Citations
TBI rehabilitation case is a case example of traumatic brain injury (TBI) using neuropsychological (NP) and neurological evaluations and follow-ups to assist the patient in helping her reach her long-term rehabilitation goals. The largest obstacles to achieving success included the patient’s defensiveness and psychological reactions to her situation, fatigue, and higher-level cognitive challenges which were detected on neuropsychological assessment.
Key Questions
What are the benefits of conducting a neuropsychological examination, and how can this data be used to help the physiatrist manage the overall care?
How can the neuropsychologist and physiatrist collaborate effectively to help the patient reach her neurorehabilitation goals?
This case study helps to demonstrate the value of NP testing in treatment of TBI, as well as the value of establishing a strong collaborative relationship between the neuropsychologist and the physiatrist specializing in brain injury rehabilitation.
This is a preview of subscription content, log in via an institution .
Buying options
- Available as PDF
- Read on any device
- Instant download
- Own it forever
- Available as EPUB and PDF
- Durable hardcover edition
- Dispatched in 3 to 5 business days
- Free shipping worldwide - see info
Tax calculation will be finalised at checkout
Purchases are for personal use only
Lezak MD, Howieson DB, Bigler ED, Tranel D. Neuropsychological assessment. New York: Oxford University Press; 2012.
Google Scholar
Brooks N, McKinlay W, Symington C, Beattie A, Campsie L. Return to work within the first seven years of severe head injury. Brain Injury. 1987;1(1):5–19.
Article CAS Google Scholar
Thomsen IV. Late outcome of very severe blunt head truma: a 10–15 year second follow-up. J Neurol Neurosurg Psychiatry. 1984;47:260–8.
Kolakowsky-Hayner SA, Miner KD, Kreutzer JS. Long-term life quality and family needs after traumatic brain injury. J Head Trauma Rehabil. 2001;16(4):374–85.
Ponsford JL, Olver JH, Curran C, Ng K. Prediction of employment status 2 years after traumatic brain injury. Brain Injury. 1995;9(1):11–20.
Borgaro SR, Baker J, Wethe JV, et al. Subjective reports of fatigue during early recovery from traumatic brain injury. J Head Trauma Rehabil. 2005;20:416–25.
Article Google Scholar
Cantor JB, Bushnik T, Cicerone K, Dijkers MP, Gordon W, Hammond FM, Kolakowsky-Hayner SA, Lequerica A, Nguyen M, Spielman LA. Insomnia, fatigue, and sleepiness in the first 2 years after traumatic brain injury: a NIDRR TBI model system module study. J Head Trauma Rehabil. 2012;27(6):E1–14.
Lucas S, Hoffman JM, Bell KR, Dikmen S. A prospective study of prevalence and characterization of headache following mild traumatic brain injury. Cephalalgia. 2014;34(2):93–102.
Godbolt AK, Stenberg M, Jakobsson J, Kimmo S, Krakau K, Stalnacke FM, DeBoussard CN. Subacute complications during recovery from severe traumatic brain injury: frequency and associations with outcome. BMJ Open. 2015;5(4):e007208.
Barnett BP, Singman EL. Vision concerns after mild traumatic brain injury. Curr Treat Options Neurol. 2015;17(2):329.
Gardani M, Morfiri E, Thomson A, O-Neill B, McMillan T. Evaluation of sleep disorders in patients with severe traumatic brain injury during rehabilitation. Arch Phys Med Rehabil. 2015;15:418–9.
Ponsford JL, Sinclair KL. Sleep and fatigue following traumatic brain injury. Psychiatr Clin North Am. 2014;37(1):77–89.
Seel RT, Kreutzer JS, Rosenthal M, Hammond FM, Corrigan JD, Black K. Depression after traumatic brain injury: a national institute on disability and rehabilitation research model systems multicenter investigation. Arch Phys Med Rehabil. 2003;84:177–84.
Deb S, Lyons I, Koutzoukis C, Ali I, McCarthy G. Rate of psychiatric Illness 1 year after traumatic brain injury. Am J Psychiatry. 1999;156:374–8.
CAS PubMed Google Scholar
Kreutzer JS, Seel RT, Gourley E. The prevalence and symptom rates of depression after traumatic brain injury: a comprehensive examination. Brain Injury. 2005;15(7):563–76.
Varney NR, Martzke JS, Roberts RJ. Major depression in patients with closed head injury. Neuropsychology. 1987;1(1):7–9.
Lezak M. Relationship between personality disorders, social disturbances, and physical disability following traumatic brain injury. J Head Trauma Rehabil. 1987;2(1):57–69.
Dickmen SS, Bombardier CH, Machamer JE, Fann JR, Temkin NR. Natural history of depression in traumatic brain injury. Arch Phys Med Rehabil. 2004;85(9):1457–64.
Jorge RE, Robinson RG, Arndt SV, Starkstein SE, Forester AW, Geisler F. Depression following traumatic brain injury: a 1 year longitudinal study. J Affect Disord. 1993;27(4):233–43.
MacNiven E, Finlayson MA. The interplay between emotional and cognitive recovery after closed head injury. Brain Injury. 1993;7:241–6.
Malec JF, Moessner AM. Self-awareness, distress, and post-acute rehabilitation outcome. Rehabil Psychol. 2000;45:227–41.
Rosenthal M, Christensen BK, Ross TP. Depression following traumatic brain injury. Arch Phys Med Rehabil. 1998;79:90–103.
Cantor JB, Ashman TA, Schwartz ME, Gordon WA, Hibbard MR, Brown M, Spielman L, Charatz HJ, Cheng Z. The role of self-discrepancy theory in understanding post-traumatic brain injury affective disorders: a pilot study. J Head Trauma Rehabil. 2005;20(6):527–43.
Lam B, Middleton LE, Masellis M, Stuss DT, Harry RD, Kiss A, Black SE. Criterion and convergent validity of the Montreal cognitive assessment with screening and standardized neuropsychological testing. J Am Geriatr Soc. 2013;61(12):2181–5.
Pendlebury ST, Markwick A, deJager CA, Zombini G, Wilcock GK, Rothwell PM. Differences in cognitive profile between TIA, stroke and elderly memory research subjects: a comparison of the MMSE and MoCA. Cerebrovasc Dis. 2012;34(1):48–54.
Breceda EY, Dromerick AW. Motor rehabilitation in stroke and traumatic brain injury: stimulating and intense. Curr Opin Neurol. 2013;26(6):595–601.
Trexler L, Webb PM, Zappala G. In: Christensen AL, Uzzell Barbara P, editors. Strategic aspects of neuropsychological rehabilitation. As seen in brain injury and neuropsychological rehabilitation: international perspectives. Hillsdale, NJ: Lawrence Erlbaum Associates, Publishers; 1994.
Galski T, Ehle HT, McDonald MA, Mackevich J. Evaluating fitness to drive after cerebral injury: basic issues and recommendations for medical and legal communities. J Head Trauma Rehabil. 2000;15:895–908.
Dawson JD, Uc EY, Anderson SW, et al. Neuropsychological predictors of driving errors in older adults. J Am Geriatr Soc. 2009;58:1090–6.
Sigurdardottir S, Andelic N, Roe C, Schanke AK. Cognitive recovery and predictors of functional outcome 1 year after traumatic brain injury. J Int Neuropsychol Soc. 2009;33:202–10.
Wilde EA, Whiteneck GG, Bogner J, Bushnik T, Cifu DX, Dikmen S, French L, Giacino JT, Hart T, Malec JF, Millis SR, Novack TA, Sherer M, Tulsky DS, Vanderploeg RD, von Steinbuechel N. Recommendations for the use of common outcome measures in traumatic brain injury research. Arch Phys Med Rehabil. 2010;91:1650–60.
Vanderploeg RD, Collins RC, Sigford B, Date E, Schwab K, Warden D. Practical and theoretical considerations in designing rehabilitation trials: the DVBIC cognitive-didactic versus functional-experiential treatment study experience. J Head Trauma Rehabil. 2006;21:179–93.
Christensen AL, Uzzell BP. International handbook of neuropsychological rehabilitation. New York: Kluwer Academic/Plenum Press; 2000.
Book Google Scholar
Parker RS. Traumatic Brain Injury and Neuropsychological Impairment: sensorimotor, cognitive, emotional, and adaptive problems of children and adults. New York: Springer-Verlag; 1990.
Mitrushina MN, Boone KB, D’Elia LF. Handbook of normative data for neuropsychological assessment. New York: Oxford University Press; 1999.
Download references
Author information
Authors and affiliations.
Department of Physical Medicine and Rehabilitation, Rehabilitation Hospital of Indiana—Indiana University, Indianapolis, IN, USA
Samantha L. Backhaus
Department of Physical Medicine and Rehabilitation, Indiana University School of Medicine, Indianapolis, IN, USA
Ana Durand-Sanchez
You can also search for this author in PubMed Google Scholar
Corresponding author
Correspondence to Samantha L. Backhaus .
Editor information
Editors and affiliations.
Department of Psychiatry and Behavioral Sciences, University of Washington School of Medicine, Seattle, WA, USA
Karen M. Sanders
Chapter Review Questions
In this case, the diagnosis of TBI was already known. Given this information, how was it useful to obtain neuropsychological testing data, and how did this guide the treatment recommendations for the patient’s outpatient treatment, particularly given that she appeared so “high-level” and obtained some “average” scores?
Why was it important to recommend that a more comprehensive neuropsychological test be given as opposed to providing a basic mental status examination for this patient? Are there times when administering mental status examinations can be useful?
Why was it important for the physiatrist to have some knowledge on treatment of mood disturbances, and when would it have been a good idea to refer out to a psychiatrist?
How does collaboration with a neuropsychologist help a physician better monitor a patient’s cognitive progress and rehabilitation process? How does it help synergize the management of mood disturbances?
Rights and permissions
Reprints and permissions
Copyright information
© 2019 Springer Science+Business Media, LLC, part of Springer Nature
About this chapter
Cite this chapter.
Backhaus, S.L., Durand-Sanchez, A. (2019). Traumatic Brain Injury Rehabilitation Case Study. In: Sanders, K. (eds) Physician's Field Guide to Neuropsychology. Springer, New York, NY. https://doi.org/10.1007/978-1-4939-8722-1_26
Download citation
DOI : https://doi.org/10.1007/978-1-4939-8722-1_26
Published : 23 May 2019
Publisher Name : Springer, New York, NY
Print ISBN : 978-1-4939-8720-7
Online ISBN : 978-1-4939-8722-1
eBook Packages : Medicine Medicine (R0)
Share this chapter
Anyone you share the following link with will be able to read this content:
Sorry, a shareable link is not currently available for this article.
Provided by the Springer Nature SharedIt content-sharing initiative
- Publish with us
Policies and ethics
- Find a journal
- Track your research
- Introduction
- Conclusions
- Article Information
eAppendix. ROC Curve Analysis
eFigure. ROC Curves of PCL-C Scores and NODDI/DTI Metrics Classifying mTBI From Control
eTable 1. Index of ROIs From the JHU-ICBM-DTI-81 WM Labels Atlas
eTable 2. Severity Ratings of GAD-7 and PHQ-9
eTable 3. Results of Sensitivity Analysis of Regression Models (TBI Only, n = 65)
eTable 4. Diagnostic Sensitivity and Specificity of PCL-C Scores and Diffusion Parameters for Classifying mTBI From Control
eReferences.
Data Sharing Statement
See More About
Sign up for emails based on your interests, select your interests.
Customize your JAMA Network experience by selecting one or more topics from the list below.
- Academic Medicine
- Acid Base, Electrolytes, Fluids
- Allergy and Clinical Immunology
- American Indian or Alaska Natives
- Anesthesiology
- Anticoagulation
- Art and Images in Psychiatry
- Artificial Intelligence
- Assisted Reproduction
- Bleeding and Transfusion
- Caring for the Critically Ill Patient
- Challenges in Clinical Electrocardiography
- Climate and Health
- Climate Change
- Clinical Challenge
- Clinical Decision Support
- Clinical Implications of Basic Neuroscience
- Clinical Pharmacy and Pharmacology
- Complementary and Alternative Medicine
- Consensus Statements
- Coronavirus (COVID-19)
- Critical Care Medicine
- Cultural Competency
- Dental Medicine
- Dermatology
- Diabetes and Endocrinology
- Diagnostic Test Interpretation
- Drug Development
- Electronic Health Records
- Emergency Medicine
- End of Life, Hospice, Palliative Care
- Environmental Health
- Equity, Diversity, and Inclusion
- Facial Plastic Surgery
- Gastroenterology and Hepatology
- Genetics and Genomics
- Genomics and Precision Health
- Global Health
- Guide to Statistics and Methods
- Hair Disorders
- Health Care Delivery Models
- Health Care Economics, Insurance, Payment
- Health Care Quality
- Health Care Reform
- Health Care Safety
- Health Care Workforce
- Health Disparities
- Health Inequities
- Health Policy
- Health Systems Science
- History of Medicine
- Hypertension
- Images in Neurology
- Implementation Science
- Infectious Diseases
- Innovations in Health Care Delivery
- JAMA Infographic
- Law and Medicine
- Leading Change
- Less is More
- LGBTQIA Medicine
- Lifestyle Behaviors
- Medical Coding
- Medical Devices and Equipment
- Medical Education
- Medical Education and Training
- Medical Journals and Publishing
- Mobile Health and Telemedicine
- Narrative Medicine
- Neuroscience and Psychiatry
- Notable Notes
- Nutrition, Obesity, Exercise
- Obstetrics and Gynecology
- Occupational Health
- Ophthalmology
- Orthopedics
- Otolaryngology
- Pain Medicine
- Palliative Care
- Pathology and Laboratory Medicine
- Patient Care
- Patient Information
- Performance Improvement
- Performance Measures
- Perioperative Care and Consultation
- Pharmacoeconomics
- Pharmacoepidemiology
- Pharmacogenetics
- Pharmacy and Clinical Pharmacology
- Physical Medicine and Rehabilitation
- Physical Therapy
- Physician Leadership
- Population Health
- Primary Care
- Professional Well-being
- Professionalism
- Psychiatry and Behavioral Health
- Public Health
- Pulmonary Medicine
- Regulatory Agencies
- Reproductive Health
- Research, Methods, Statistics
- Resuscitation
- Rheumatology
- Risk Management
- Scientific Discovery and the Future of Medicine
- Shared Decision Making and Communication
- Sleep Medicine
- Sports Medicine
- Stem Cell Transplantation
- Substance Use and Addiction Medicine
- Surgical Innovation
- Surgical Pearls
- Teachable Moment
- Technology and Finance
- The Art of JAMA
- The Arts and Medicine
- The Rational Clinical Examination
- Tobacco and e-Cigarettes
- Translational Medicine
- Trauma and Injury
- Treatment Adherence
- Ultrasonography
- Users' Guide to the Medical Literature
- Vaccination
- Venous Thromboembolism
- Veterans Health
- Women's Health
- Workflow and Process
- Wound Care, Infection, Healing
Get the latest research based on your areas of interest.
Others also liked.
- Download PDF
- X Facebook More LinkedIn
Kim S , Ollinger J , Song C, et al. White Matter Alterations in Military Service Members With Remote Mild Traumatic Brain Injury. JAMA Netw Open. 2024;7(4):e248121. doi:10.1001/jamanetworkopen.2024.8121
Manage citations:
© 2024
- Permissions
White Matter Alterations in Military Service Members With Remote Mild Traumatic Brain Injury
- 1 Program in Neuroscience, Uniformed Services University of Health Sciences, Bethesda, Maryland
- 2 School of Medicine, Uniformed Services University of Health Sciences, Bethesda, Maryland
- 3 National Intrepid Center of Excellence, Walter Reed National Military Medical Center, Bethesda, Maryland
- 4 Department of Preventive Medicine and Biostatistics, Uniformed Services University of Health Sciences, Bethesda, Maryland
- 5 Department of Psychiatry, Yale University School of Medicine, New Haven, Connecticut
- 6 Directorate of Behavioral Health, Walter Reed National Military Medical Center, Bethesda, Maryland
- 7 Department of Neurology, Walter Reed National Military Medical Center, Bethesda, Maryland
Question Can the use of diffusion tensor imaging and neurite orientation dispersion and density imaging effectively detect white matter microstructural alterations in US military service members with a history of mild traumatic brain injury occurring more than 2 years ago, and can the results be used in monitoring neurobehavioral symptoms?
Findings In this case-control study of 98 military service members, both imaging tools detected widespread differences in white matter, with more sensitive results occurring with the use of neurite orientation dispersion and density imaging metrics through a region-of-interest approach. Notably, these white matter alterations were found to be associated with neurobehavioral symptoms.
Meaning These findings support the usefulness of advanced neuroimaging techniques in assessing microstructural changes related to military-related mild traumatic brain injury, and suggest that aberrant white matter properties can be used in monitoring progression or recovery during the chronic postinjury phase.
Importance Mild traumatic brain injury (mTBI) is the signature injury experienced by military service members and is associated with poor neuropsychiatric outcomes. Yet, there is a lack of reliable clinical tools for mTBI diagnosis and prognosis.
Objective To examine the white matter microstructure and neuropsychiatric outcomes of service members with a remote history of mTBI (ie, mTBI that occurred over 2 years ago) using diffusion tensor imaging (DTI) and neurite orientation dispersion and density imaging (NODDI).
Design, Setting, and Participants This case-control study examined 98 male service members enrolled in a study at the National Intrepid Center of Excellence. Eligible participants were active duty status or able to enroll in the Defense Enrollment Eligibility Reporting system, ages 18 to 60 years, and had a remote history of mTBI; controls were matched by age.
Exposures Remote history of mTBI.
Main Outcomes and Measures White matter microstructure was assessed using a region-of-interest approach of skeletonized diffusion images, including DTI (fractional anisotropy, mean diffusivity, radial diffusivity and axial diffusivity) and NODDI (orientation dispersion index [ODI], isotropic volume fraction, intra-cellular volume fraction). Neuropsychiatric outcomes associated with posttraumatic stress disorder (PTSD) and postconcussion syndrome were assessed.
Results A total of 65 male patients with a remote history of mTBI (mean [SD] age, 40.5 [5.0] years) and 33 age-matched male controls (mean [SD] age, 38.9 [5.6] years) were included in analysis. Compared with the control cohort, the 65 service members with mTBI presented with significantly more severe PTSD-like symptoms (mean [SD] PTSD CheckList-Civilian [PCL-C] version scores: control, 19.0 [3.8] vs mTBI, 41.2 [11.6]; P < .001). DTI and NODDI metrics were altered in the mTBI group compared with the control, including intra-cellular volume fraction of the right cortico-spinal tract (β = −0.029, Cohen d = 0.66; P < .001), ODI of the left posterior thalamic radiation (β = −0.006, Cohen d = 0.55; P < .001), and ODI of the left uncinate fasciculus (β = 0.013, Cohen d = 0.61; P < .001). In service members with mTBI, fractional anisotropy of the left uncinate fasciculus was associated with postconcussion syndrome (β = 5.4 × 10 −3 ; P = .003), isotropic volume fraction of the genu of the corpus callosum with PCL-C (β = 4.3 × 10 −4 ; P = .01), and ODI of the left fornix and stria terminalis with PCL-C avoidance scores (β = 1.2 × 10 −3 ; P = .02).
Conclusions and Relevance In this case-control study of military-related mTBI, the results suggest that advanced magnetic resonance imaging techniques using NODDI can reveal white matter microstructural alterations associated with neuropsychiatric symptoms in the chronic phase of mTBI. Diffusion trends observed throughout widespread white matter regions-of-interest may reflect mechanisms of neurodegeneration as well as postinjury tissue scarring and reorganization.
Traumatic brain injury (TBI) is a significant health concern, particularly among military populations. According to the Defense and Veterans Brain Injury Center (DVBIC), more than 450 000 TBIs among US service members worldwide have been reported between 2000 and 2022, with over 80% of those TBIs being classified as mild (mTBI). 1 Mounting evidence has demonstrated the neuropsychiatric consequences of mTBI, including chronic postconcussion symptoms and serious long-term effects on cognition, memory, mood, sleep, vision, and hearing. 2 - 4 Furthermore, individuals with a history of mTBI are at higher risk for dementia, neurodegenerative diseases, psychiatric illness, and even mortality, indicating long-term progression of subclinical pathology that can manifest later in life. 5 - 7
Despite mTBI lacking obvious clinical neuroimaging findings, making the detection of postinjury neurological changes challenging, there is increasing recognition that advanced neuroimaging techniques are promising biomarkers for its diagnosis, prognosis, and treatment monitoring. 8 , 9 Specifically, diffusion-weighted imaging (DWI) utilizes the diffusion of water within brain tissue to infer microstructural tissue properties. One such advanced technique is diffusion tensor imaging (DTI), which provides a measure of the microstructural integrity of white matter fiber tracts through modeling of the DWI data sets. Within each voxel, DTI estimates specific diffusion variables, including mean diffusivity, fractional anisotropy, axial diffusivity, and radial diffusivity ( Table 1 ). 29 , 30
Previous studies have demonstrated that white matter microstructural integrity, measured by DTI metrics, is perturbed following military-related mTBI. 31 - 35 Additionally, DTI studies of mTBI have reported that microstructural white matter disruptions are associated with neurocognitive and behavioral deficits postinjury. 36 - 38 However, studies of military mTBI have generally yielded varied findings on which white matter tracts are affected and whether fractional anisotropy is increased or decreased following injury. 39 For instance, some studies report lower fractional anisotropy after remote mTBI, 40 , 41 elevated fractional anisotropy, 42 or a lack of significant mTBI effects on fractional anisotropy. 31 , 43 - 46 These inconsistencies may be attributed to the variability in the mechanism (ie, different cellular alterations) and etiology of mTBI at different time points postinjury.
DTI metrics represent basic mathematical descriptions of diffusion that lack structural specificity and do not directly correspond to biophysically meaningful parameters of the underlying tissue. DTI assumes Gaussian diffusion within a single microstructural compartment and is thus insensitive to the complexity of white matter microstructure that is depicted through non-Gaussian models with multiple compartments. 47 To address this limitation, a neurite orientation dispersion and density imaging (NODDI) model was created to offer more specific indices of tissue microstructure. 48 NODDI leverages recent progress in high-performance magnetic field gradients for magnetic resonance imaging (MRI) scanners that can more specifically probe complex non-Gaussian properties of white matter diffusion ( Table 1 ). A 2022 study 22 on civilian subjects used NODDI to identify longitudinal white matter changes of declining neurite density after mTBI, suggesting axonal degeneration from diffuse axonal injury. The study concluded that NODDI metrics are more sensitive and specific biomarkers than DTI for white matter microstructural changes.
However, further investigation using both DTI and NODDI is needed in military populations to improve the health and well-being of service members at higher risk of TBIs and their associated consequences. To our knowledge, this case-control study is the first to leverage DTI and NODDI to examine subtle white matter neuropathological changes in military service members with mTBI as well as their association with neuropsychiatric symptomology in the chronic phase postinjury. We hypothesize that NODDI would be more sensitive and specific to microstructural changes than DTI and that diffusion metrics would be associated with neuropsychiatric symptoms. By combining advanced neuroimaging techniques and neuropsychiatric data, we aim to gain deeper insights into the underlying brain changes associated with military mTBI, ultimately enhancing our understanding and management of this complex condition.
All participants were US military service members enrolled in the Walter Reed National Military Medical Center institutional review board–approved and HIPAA-compliant Neuroimaging Core project. All participants gave written informed consent. This cross-sectional study followed the Strengthening the Reporting of Observational Studies in Epidemiology ( STROBE ) reporting guideline.
Participants were scanned at the National Intrepid Center of Excellence at the Walter Reed National Military Medical Center. Inclusion criteria include active duty status or eligibility for the Defense Enrollment Eligibility Reporting system, age 18 to 60 years, male sex or female sex with no current pregnancy or breastfeeding. Exclusion criteria included patients with TBI unable to consent, actively enrolled in other treatment trials where this study would interfere, a history of prior severe neurologic or psychiatric conditions unrelated to the injury event or deployment (eg, meningioma, bipolar disorder), and patients with metal implants or shrapnel. Patients were targeted for recruitment and consent to the study if they had a history of mTBI (ie, mTBI group) or had no mTBI (ie, noninjured controls). Individuals were included in the mTBI group based on confirmed mTBI diagnosis with a history exceeding 2 years, male sex, and active duty status. Participants in the control group were matched for age, sex, and active duty status. Participants from both groups were excluded if they had incomplete PCL-C survey, DTI, or NODDI data. Diagnosis of TBI is described in eMethods in Supplement 1 .
The assessment of commonly affected neuropsychiatric domains after mTBI involved self-report surveys administered prior to the scan. These surveys included the PTSD Checklist–Civilian version (PCLC-C), 49 Neurobehavioral Symptom Inventory (NSI), 50 Generalized Anxiety Disorder-7 (GAD-7), 51 and Patient Health Questionnaire-9 (PHQ-9). 52 The PCL-C is a 17-item measure modeled after the Diagnostic and Statistical Manual of Mental Disorders (Fourth Edition, Text Revision) ( DSM-IV-TR ) 53 symptom criteria for PTSD. Three cluster scores corresponding to the DSM-IV-TR symptom criteria were calculated: criterion B (reexperiencing cluster), criterion C (avoidance cluster), and criterion D (hyperarousal cluster). The NSI is a 22-item measure designed to evaluate self-reported postconcussion symptoms, such as headache, balance issues, and nausea, rated on a 5-point scale. A total score was obtained by summing the ratings for the 22 items, and 4 cluster scores were calculated as outlined by Vanderploeg and colleagues 54 : vestibular, somatosensory, cognitive, and affective clusters. The GAD-7 is a self-report survey consisting of 7 items designed to assess the severity of generalized anxiety disorder symptoms in individuals over the past 2 weeks. The PHQ-9 is a self-report questionnaire comprising 9 items used to measure the severity of depression symptoms and aid in diagnosing depressive disorders. While participants in both the mTBI and control groups completed the PCL-C, only patients with mTBI completed the NSI, GAD-7, and PHQ-9 surveys.
All subjects were scanned on a 3T MR750 scanner (General Electric) equipped with a 32-channel phased array head radiofrequency coil (MR Instruments). Whole-brain diffusion and structural MRI were obtained. Head motion was represented by framewise displacement measures of diffusion MRI (dMRI) 55 and images were preprocessed with the protocol discussed in eMethods in Supplement 1 .
DTI scalar images (ie, fractional anisotropy, mean diffusivity, axial diffusivity, and radial diffusivity) were created from dMRI with b-values between 0 and 1000 seconds/mm 2 using the log-signal in 2 steps implemented in MRtrix3. 56 Weighted least-squares with weights based on the empirical signal intensity followed by iterated weighted least-squares with weights determined by the signal predictions from the previous iteration using unconstrained optimization were applied to reconstructed DTI maps. NODDI parameters were calculated using the open-source tool AMICO, 57 which yielded maps of intracellular volume fraction (ICVF), orientation dispersion index (ODI), and isotropic volume fraction (ISOVF) for each participant. 48
In a region-of-interest (ROI) analysis, the fractional anisotropy data were warped to the common FMRIB58 fractional anisotropy template in MNI152 standard space using the symmetric image normalization method implemented in the ANTs package 58 using FMRIB Software Library’s toolbox tract-based spatial statistics (TBSS), a mean fractional anisotropy image was generated from all participant scans in this common space, creating a mean fractional anisotropy white matter skeleton representing common tracts across the entire group and thresholded at above 0.2 to exclude voxels containing gray matter and partial volume effects. The aligned fractional anisotropy volume was projected onto the skeleton by filling it with fractional anisotropy values from the nearest relevant tract center. Output images and the 0.2-thresholded skeleton were visually inspected for accuracy. The same nonlinear warping and skeleton projection steps were then applied to other whole brain mean diffusivity, radial diffusivity, axial diffusivity, ICVF, ODI, and ISOVF maps.
White matter main ROIs were examined by utilizing the Johns Hopkins University (JHU) ICBM-DTI-81 white matter Labeled Atlas 59 in the standard MNI152 space. The JHU-ICBM-DTI-81 white matter labels atlas contains 46 white matter labels (eTable 1 in Supplement 1 ). To analyze these fasciculi, binary mask images corresponding to each tract were used to mask individual skeletonized maps that had been previously registered to the MNI (Montreal Neurological Institute) standard space. Regional values represented by the average voxel value within the selected JHU white matter tract masks were computed for each participant across all generated DTI and NODDI parameter maps.
All statistical analyses were performed using SPSS Statistics version 24.0 (IBM Corp) and R package 4.2.2 (R Project for Statistical Computing). Unpaired 2-sample t tests were used for demographic analysis between groups. χ 2 tests compared the proportion of counts in each military-related category between groups with the expected proportions and the null hypothesis of equal proportions in each group. To compare DTI and NODDI parameters between mTBI and control groups, a generalized linear model (GLM) analysis was conducted with covariates of age and mean framewise displacement followed by nonparametric permutation test and randomization test (eMethods in Supplement 1 ). Variance inflation factor (VIF) was computed to assess the severity of multicollinearity in the OLS regression analysis. 60 Finally, we performed a sensitivity analysis by comparing the variances between full and reduced OLS models and calculating the standardized regression coefficients (SRC), a global sensitivity indices based on linear or monotonic assumptions in the case of independent factors, 61 , 62 and the Johnson indices, indices for correlated input relative importance by R 2 decomposition for linear regression models (eMethods in Supplement 1 ). 63 , 64 The dichotomous receiver operating characteristic (ROC) curve analysis is described in eAppendix in Supplement 1 . A 2-tailed P value at .05 was considered statistically significant.
A total of 98 study participants were included (all male; mean [SD] age, 40.0 [5.2] years); 33 participants had no history of mTBI (mean [SD] age, 39.1 [5.6] years) and 65 participants had a history of mTBI (mean [SD]age, 40.6 [5.0] years) ( Table 2 ). Participants with a history of mTBI had significantly fewer education years compared with those in the control group (mean [SD] education, 14.7 [2.2] years vs 16.3 [2.8] years; P = .006). A significantly greater proportion of participants in the control group were in the Army compared with those in the mTBI group (22 of 33 [67%] vs 15 of 65 [23%]; P < .001). A significantly higher proportion of participants with mTBI were enlisted (53 of 65 [82%] vs 17 of 33 [52%]; P = .002). The mean (SD) mTBI count was 2.1 (1.4), with a mean (SD) time since the most recent injury being 14.2 (9.3) years. Most of the mechanisms causing mTBI were attributed to impacts (24 [37%]) and falls (20 [31%]). Additionally, 9 injuries (14%) resulted from blasts, 10 (15%) from motor vehicle accidents, 1 (2%) from gunshot wounds, and 1 (2%) from various other causes.
Compared with the control group, participants with mTBI history had significantly higher PCL-C total scores (mean [SD] score, 40.9 [11.3] vs 19.0 [3.8]; P < .001). The mTBI group had a mean (SD) NSI total score of 36.1 (12.4), a somatosensory subscore of 8.4 (4.4), an affective subscore of 12.1 (4.6), a cognitive subscore of 9.1 (3.0), and a vestibular subscore of 3.4 (2.0). The majority of participants with mTBI history had moderately severe (20 participants [31%]) and severe (20 [31%]) anxiety as indicated by the GAD-7 scores as well as moderate (25 [39%]), moderately severe (6 [9%]), and severe (4 [6%]) depression as indicated by the PHQ-9 scores (eTable 2 in Supplement 1 ). Three service members with mTBI (4.6%) did not have NSI scores, which were replaced by imputation using R MICE. NSI, GAD-7, and PHQ-9 were not obtained in healthy controls.
There was no significant group difference of dMRI framewise displacement. This suggests participants with mTBI history had no greater head motion than controls during MRI examination.
ROI analyses using GLMs with age as a covariate revealed widespread differences in DTI and NODDI metrics in various white matter regions ( Table 3 ). Notably, more NODDI metrics were significantly different between control and mTBI groups compared with DTI metrics. Diffusion metrics of ROIs with the highest effect sizes between mTBI and control groups included ICVF of the right corticospinal tract (CST) (β = −0.029, R 2 = 0.136; P < .001), ODI of the left posterior thalamic radiation (PTR) (β = −6 × 10 −3 , R 2 = 0.253; P < .001) and ODI of the left uncinate fasciculus (UNC) (β = 0.013, R 2 = 0.125; P < .001).
When assessing the association with neuropsychiatric symptoms, NSI cognitive subscores were associated with fractional anisotropy of the left UNC (β = 5.4 × 10 −3 ; P = .003); PCL-C total scores were associated with ISOVF of the genu of corpus callosum (β = 4.3 × 10 −4 ; P = .01); PCL-C C avoidance subscores were associated with ODI of the left fornix (crus) and stria terminalis (β = 1.2 × 10 −3 ; P = .02). VIF, an index measuring how much the variance of an estimated regression coefficient is increased because of collinearity, of all models were less than 1.2 ( Table 4 ). Sensitivity analysis of the 3 regression models revealed that the full model (3 independent variables) explained the variances of diffusion metrics better than those of the reduced model (2 independent variables) with an SRC of 0.335, 0.266, and 1.000, and R 2 of 0.091, 0.067, and 0.867 (Johnson indices) for NSI cognitive, PCL-C total scores and PCL-C C avoidance subscores, respectively (eTable 4 in Supplement 1 ). ROC curve analysis is available in eResults in Supplement 1 .
This case-control study investigated diffusion parameters of white matter in military service members with and without remote mTBI. To the best of our knowledge, this is the first study to report white matter microstructural changes indicated by DTI and NODDI metrics using an ROI-based approach in the chronic phase of mTBI among military service members. Our study supports previous evidence that mTBI can have long-term effects on white matter microstructure and neuropsychiatric symptoms related to PTSD, postconcussion syndrome, anxiety, and depression. 65 - 68
The ROI analysis yielded extensive white matter alterations in the mTBI group that were also linked to neuropsychiatric symptoms. Notably, the analysis revealed DTI and NODDI trends of increased anisotropic and parallel diffusion, implicating mechanisms of inflammation, glial cell activation, and tissue scarring or reorganization. 69 , 70 For example, there was increased fractional anisotropy and axial diffusivity, as well as decreased ODI in the left PTR. It is often assumed that lower fractional anisotropy values may correspond to a reduction of the white matter microstructural integrity. 71 However, emerging evidence suggests that higher fractional anisotropy and axial diffusivity values can be attributed to factors such as cytotoxic edema during the acute phase following injury 72 - 74 as well as glial scarring and a manifestation of recovery and/or compensation in the chronic phase, 69 , 72 , 74 - 76 or simply in the voxels with less fiber crossings. In these conditions, the interpretation of higher fractional anisotropy values as solely indicative of increased white matter integrity may not hold true. Some studies indicated that astrocytes undergoing structural remodeling postinjury, thereby driving glial scarring, lead to anisotropic tissue microstructure causing an increase in fractional anisotropy. 69 , 70 , 77 Notably, a 2011 study 69 performing a histological analysis showed that glial fibrillary acidic protein (GFAP), which is a marker for gliosis, was significantly positively correlated with fractional anisotropy and axial diffusivity. Furthermore, decreased mean diffusivity, radial diffusivity, ISOVF, and ODI were observed in the fornix, with ODI values being associated with PCL-C scores. Reduced ISOVF in the corpus callosum was also associated with PCL-C total. Altogether, our findings of compromised white matter in these key tracts complement previous findings that also showed white matter changes in military service members with a remote history of mTBI. 41 , 78
Alternatively, our study also revealed diffusion trends implicating mechanisms of neurodegeneration. For example, decreased ICVF in right and left CST suggests reduced neurite density; reduced fractional anisotropy with increased ODI in the left UNC may suggest axonal degeneration with compensatory neural sprouting. 79 - 81 These findings are consistent with our 2023 study 82 investigating fiber-specific structural changes in a larger cohort of military service members after a remote brain injury. Importantly, fractional anisotropy was significantly reduced in the left UNC and was associated with cognitive-related PCS scores. This anatomically aligns with the significance of UNC in key neural circuitry involving the entorhinal and amygdala, which plays a pivotal role in memory formation and emotion regulation. 83 , 84 Postconcussion symptoms, PTSD symptoms, and neuropsychological function have been shown to be associated with compromised fronto-limbic neurocircuitry in chronic mTBI. 85 , 86
While statistical significance shows that an association exists in a study, effect size indicates the practical significance of a research outcome. R 2 determines the proportion of variance in the dependent variable that can be explained by the independent variable, representing the goodness of fit. Our results show that the ICVF of the right CST had a high Cohen d for group difference but a low R 2 value, while the ODI of left fornix and stria terminalis had a high SRC and R 2 but a relatively low Cohen d . These findings might help explain why diffusion metrics exhibit low dichotomous discrimination.
Lastly, ROC curve analyses indicated that self-reported neuropsychiatric symptoms were more effective in distinguishing between the mTBI and control groups compared with imaging metrics, which did not provide significant discriminatory power. Particularly, PTSD symptoms were successful in classifying participants with mTBI history from controls in this patient population. Prior studies have highlighted the increased risk of comorbid PTSD and mTBI among military service members compared with civilians. 87 - 90 This emphasizes the importance of assessing PTSD symptoms and associated neuropsychiatric symptoms in the management of military-related mTBI. Indeed, while neuroimaging metrics did not show superior discriminatory ability, they did facilitate the identification of potential lesions associated with neuropsychiatric outcomes, which can be used to predict clinical progression and determine the most suitable treatment approach. 91 , 92
This study had several limitations. This was a cross-sectional case-control study with a relatively limited sample size, which offers only a snapshot of the neural factors associated with neuropsychiatric symptoms in the chronic phase postinjury. Although the sample size was similar to other studies using NODDI to examine mTBI, 22 , 23 , 28 , 93 , 94 further analysis with a larger sample is necessary to validate the findings. Additionally, there were missing clinical data that might have influenced the results, including past medical history, preexisting conditions, medications, treatments, or interventions received. Finally, a causal relationship between white matter microstructural alterations and neuropsychiatric symptom presentation cannot be assumed. Thus, these findings should be considered as suggestive of a relationship that should be replicated in other studies.
In this case-control study of military-related mTBI, our results showed that DTI and NODDI can detect microstructural white matter alterations in the chronic phase of mTBI, implicating inflammatory and neurodegenerative processes. Moreover, our findings suggest that NODDI might offer greater sensitivity than DTI in identifying alterations during the chronic phase. Consequently, combining NODDI with DTI parameters in future research could yield valuable insights. Specifically, our results suggest that mTBI in military service members is characterized by widespread differences in diffusion parameters of white matter tracts important for cognitive and emotional processing, including the corpus callosum, CST, UNC, and fornix. Our findings indicate that DTI and NODDI metrics can provide valuable pathophysiological insights into the long-term neuropsychiatric consequences of mTBI.
Accepted for Publication: February 25, 2024.
Published: April 18, 2024. doi:10.1001/jamanetworkopen.2024.8121
Open Access: This is an open access article distributed under the terms of the CC-BY License . © 2024 Kim S et al. JAMA Network Open .
Corresponding Author: Ping-Hong Yeh, PhD, RM1128, Bldg 51, National Intrepid Center of Excellence (NICoE), Walter Reed National Military Medical Center, Bethesda, MD 20914 ( [email protected] ; [email protected] ).
Author Contributions: Drs Kim and Yeh had full access to all of the data in the study and takes responsibility for the integrity of the data and the accuracy of the data analysis. Drs Kim and Yeh contributed equally to this article.
Concept and design: Kim, Ollinger, Yeh.
Acquisition, analysis, or interpretation of data: Kim, Song, Raiciulescu, Seenivasan, Wolfgang, Werner, Yeh.
Drafting of the manuscript: Kim, Yeh.
Critical review of the manuscript for important intellectual content: All authors.
Statistical analysis: Kim, Ollinger, Raiciulescu, Yeh.
Administrative, technical, or material support: Song, Seenivasan, Yeh.
Supervision: Ollinger, Wolfgang, Werner, Yeh.
Conflict of Interest Disclosures: None reported.
Funding/Support: This project was funded and partly supported by US Army Medical Research and Materiel Command (USAMRMC) (award No. 203337).
Role of the Funder/Sponsor: The institutions with which the authors of this study are affiliated, including USAMRMC, National Intrepid Center of Excellence, Walter Reed National Military Medical Center, and Uniformed Services University of Health Sciences, played no part in the design or conduct of the study; collection, management, analysis, interpretation of the data, manuscript preparation, nor the decision to submit the manuscript for publication. The manuscript was reviewed and approved by the public affairs official at the Uniformed Services University of Health Sciences.
Disclaimer: The opinions and assertions expressed herein are those of the author(s) and do not reflect the official policy or position of the Uniformed Services University of the Health Sciences or the Department of Defense.
Data Sharing Statement: See Supplement 2 .
Additional Contributions: The authors would like to acknowledge the efforts of the larger team of research coordinators, technical support, and senior management at the Neuroimaging Section of the Research Department, National Intrepid Center of Excellence (NICoE). Particular thanks to Dr Rujirutana Srikanchanat, PhD, Dr Cheng Guan Koay, PhD, Dr Wei Liu, PhD, Mr Adam Cliffton, BA, Mr Joseph Hennesy, BA, and Ms Rebecca Sandlain, BA, for their assistance in MRI data acquisition (affiliated with NICoE); Dr Gerard Riedy, MD, PhD, Dr Grant Bonavia, MD, PhD, and Dr Treven Pickett, PsyD, for administrative support (affiliated with NICoE); Dr Kimbra Kenney, MD, and Dr Chandler Rhodes, PhD, for administering OSU-TBI identification methods (affiliated with NICoE); Dr Hosung Kim, PhD (affiliated with University of South California), for his discussion of the manuscript, and all the clinicians at the NICoE for their hard work of administering interview and clinical care to all the service member participants. None of the contributors has received any compensation beyond terms of employment.
- Register for email alerts with links to free full-text articles
- Access PDFs of free articles
- Manage your interests
- Save searches and receive search alerts
We have a new app!
Take the Access library with you wherever you go—easy access to books, videos, images, podcasts, personalized features, and more.
Download the Access App here: iOS and Android . Learn more here!
- Remote Access
- Save figures into PowerPoint
- Download tables as PDFs

Case Study 1: Patient With Traumatic Brain Injury
Temple T. Cowden
- Download Chapter PDF
Disclaimer: These citations have been automatically generated based on the information we have and it may not be 100% accurate. Please consult the latest official manual style if you have any questions regarding the format accuracy.
Download citation file:
- Search Book
Jump to a Section
Examination.
- Systems Review
- Tests and Measures
- Evaluation, Diagnosis and Prognosis, and Plan of Care
- Video Summary
- Full Chapter
- Supplementary Content
Demographic Information:
Patient is a 41-year-old, Filipino-Caucasian man. He is English speaking and right-hand dominant. He was admitted to the adult brain injury service for inpatient rehabilitation following a motorcycle accident.
Social History:
Patient is an ex-Marine; he has one son and is divorced from his wife. He lives with his fiancée in a single-story home. His fiancée has three children who live with them, ages 12, 13, and 15. The patient's fiancée does not work outside of the home but is responsible for the cooking, laundry, cleaning, and upkeep of the home, as well as taking care of the children.
Employment:
Patient worked as an inspector for a fire safety company. His job involved driving to various locations where he inspected and tested various fire safety equipment (such as fire alarms, fire extinguishers, and so forth), which involved a moderate amount of physical activity.
Living Environment:
Patient resides in a single-story home, which is split level, with one step to enter the home's main living room area. There are three steps to enter the home, with no handrails or ramp in place.
General Health Status:
Patient is medically stable and able to participate in a rehabilitation program. Prior to this injury, patient was believed to be in good general health.
Social and Health Habits:
Patient enjoys playing basketball and softball with his friends. Prior to injury, he was not involved in a formal exercise program but remained active playing sports, spending time with the children and through work activities. Patient states his favorite activities are riding motorcycles and "hanging out at the bars." While it is unclear how much, the patient has a history of alcohol use; his use of other drugs is unknown.
Medical/Surgical History:
Medical history is unknown due to incomplete medical records from previous facility. Patient is agitated and unable to consistently and appropriately answer questions. Surgical history prior to this injury is unknown.
History of Present Illness:
Pop-up div Successfully Displayed
This div only appears when the trigger link is hovered over. Otherwise it is hidden from view.
Please Wait
MINI REVIEW article
The emerging importance of skull-brain interactions in traumatic brain injury.

- Department of Neurology, McGovern Medical School, The University of Texas Health Science Center at Houston, Houston, TX, United States
The recent identification of skull bone marrow as a reactive hematopoietic niche that can contribute to and direct leukocyte trafficking into the meninges and brain has transformed our view of this bone structure from a solid, protective casing to a living, dynamic tissue poised to modulate brain homeostasis and neuroinflammation. This emerging concept may be highly relevant to injuries that directly impact the skull such as in traumatic brain injury (TBI). From mild concussion to severe contusion with skull fracturing, the bone marrow response of this local myeloid cell reservoir has the potential to impact not just the acute inflammatory response in the brain, but also the remodeling of the calvarium itself, influencing its response to future head impacts. If we borrow understanding from recent discoveries in other CNS immunological niches and extend them to this nascent, but growing, subfield of neuroimmunology, it is not unreasonable to consider the hematopoietic compartment in the skull may similarly play an important role in health, aging, and neurodegenerative disease following TBI. This literature review briefly summarizes the traditional role of the skull in TBI and offers some additional insights into skull-brain interactions and their potential role in affecting secondary neuroinflammation and injury outcomes.
Introduction
Traumatic Brain Injury (TBI) plays a significant role in morbidity and mortality within the United States, encompassing a range of injuries affecting brain function due to an external force to the skull ( 1 ). Approximately 2.8 million head injuries occur each year in the United States alone, with skull fracture occurring in 28-37% of those diagnosed with TBI ( 2 , 3 ). The burden of TBI is significant, with fatalities associated with TBI estimated to number more than 69,000 annually and 3.2-5.3 million individuals suffering from lasting disability in the US ( 4 , 5 ). TBI predominates among men, young children, and elderly populations, with incidence rates influenced by socioeconomic factors ( 6 ). Following either penetrating or blunt, non-penetrating traumas, TBI leads to symptoms such as altered consciousness, amnesia, confusion, headache, or dizziness ( 7 ). Severe complications include intracranial hemorrhage (ICH), heightened intracranial pressure (ICP), brain edema, and seizures ( 8 ). The initial trauma is often termed the primary injury, leading to the secondary injury or the pathophysiological responses to trauma ( 9 ). The secondary injury includes neuroinflammatory processes, excitotoxicity, and eventual cell death ( 10 ). Current treatment strategies for TBI primarily focus on the secondary injury mechanisms following brain injury. Most of these involve relieving elevated ICP via surgical intervention, anti-inflammatory medication, cranial temperature modulation, or elevation of the head ( 11 ).
Penetrating TBI involves foreign object intrusion into brain tissue, often due to injuries caused by projectiles like gunshot wounds ( 7 ). In contrast, blunt, non-penetrating TBI results from direct head impact or rapid head acceleration and deceleration without direct contact ( 7 ). Blunt non-penetrating TBI frequently leads to a contrecoup brain injury, causing brain contusion on the opposite side to the external force ( 12 ). This phenomenon arises due to the sudden movement of denser cerebrospinal fluid (CSF) towards the skull impact point, displacing the brain towards the opposite side of the skull and causing the contrecoup injury ( 13 ). The health and structural integrity of the skull as a living tissue, is thus directly and critically involved in determining the severity of TBI. However, until recently, the involvement of skull bone marrow cells, including hematopoietic cells, bone-modifying osteocytes, and other stromal cells, has been long overshadowed by the evolving neuropathology seen in the brain.
The International Classification of Diseases, Tenth Revision, Clinical Modification (ICD-10-CM) diagnostic codes for TBI include skull fracturing ( 7 ). However, TBI severity is typically classified as mild, moderate, or severe based on the Glasgow Coma Scale (GCS), which evaluates eye-opening, motor, and verbal responses ( 14 ). The GCS does not account for skull fracturing in TBI. Notably, skull fractures are evident in approximately 5% of mild TBI cases and up to 50% of severe TBI cases, correlating with worse TBI prognoses ( 2 , 15 ). Non-penetrating TBI accounts for 90% of skull fractures, with the remaining 10% originating from penetrating TBI ( 16 ). Disparities between these two evaluations, and more, may result in improper treatment plans and poorer outcomes.
While research has extensively explored the secondary brain injury following TBI, the biological role of the skull in mitigating or exacerbating the pathophysiological response remains unclear. Initially regarded as a static protective structure for the brain and meninges, recent studies have uncovered a significant role for the skull bone marrow (BM) as a distinctive site housing immune cells crucial for sensing and participating in central nervous system (CNS) inflammation ( 17 ). Moreover, direct connections between the skull and brain allow skull BM-derived immune cells to migrate into brain tissue after injury ( 18 ). This review aims to synthesize current literature on experimental TBI models, the inflammatory response post-TBI, and the skull’s involvement in TBI. Furthermore, we aim to evolve the view of the skull as not just an inert protective casing for the brain, but as a dynamic tissue that is actively involved in the brain’s response to trauma.
The skull and TBI
The human neurocranium is a composite bone structure composed of two compact cranial tables enclosing a cancellous intermediary layer of the skull, termed the diploe ( 19 ). Importantly for the discussion of brain-skull immune dynamics, the diploe serves as the primary reservoir of skull-derived bone marrow. Studies investigating the relative force capacity of each of the skull’s layers have found that it is this composite sandwich structure that accounts for the human skull’s impressive durability, rather than the strength of any individual layer ( 19 ). As skull thickness may be a significant protective modifier in TBI and skull fracture risk, apart from impact velocity, one of the most important predictors of blunt force TBI severity is impact location. Bone thickness within the cranium ranges significantly, even within the same individual bone plate ( 19 ). Unlike other bone structures, cranium bone thickness does not appear to decrease in an age-dependent manner, but it does exhibit the same trend of reduction in flexibility ( 20 ). Currently, the interaction between age and sex on cranial thickness and strength remains unclear ( 20 ). It is worth noting here that while skull fracture can occur in severe TBI and contribute to post-injury fragility, moderate TBI has been shown to cause an increase in cranial thickness which may be protective against cranial fracture in cases of repeated TBI ( 21 ).
Furthermore, while the skull serves an invaluable protective role against the mechanical forces that result in brain injury, it also contributes to TBI through the contact forces imposed on the brain during coup and contrecoup injury, as well as through the sheering forces induced between superficial brain areas and the bony protrusions of the frontal and temporal internal cranial fossae ( 22 ). Although the direct impact of the brain within the cranial vault mediates primary injury, the skull also provides a means for therapeutic intervention via craniotomy to release ICP. Additionally, the vascular channels acting as an interface between the skull and meninges have been proposed as a potentially novel route of drug delivery across the BBB, with intraosseous administration of drug compounds reaching 10- to 100-fold greater penetration into the brain parenchyma compared to systemic administration ( 23 ). Emerging data also indicate that skull bone health may be critical for normal brain function, especially in the context of repetitive mild concussion or age-specific TBI ( 24 ). Skull-targeted therapies may be in the nascent phase of preclinical development but have strong potential to attenuate the local inflammatory environment or prevent bone fracturing and dural bleeding.
Acute inflammatory response to TBI
During the secondary phase of injury, there are numerous mechanisms to promote the inflammatory response following TBI, including apoptotic cascades, reactive oxygen species (ROS) generation, increased BBB permeability, and mitochondrial dysfunction ( 25 – 27 ). Damage-Associated Molecular Patterns (DAMPs) like ATP, Heat-Shock Proteins (HSPs), and High Mobility Group Box 1 (HMGB1) are released from neurons and glial cells, activating innate immune receptors on macrophages, dendritic cells, and glial cells ( 28 ). This activation prompts microglia, the resident macrophages of the CNS, to clear debris and intensify inflammation by releasing pro-inflammatory cytokines ( 29 , 30 ). These cytokines, including IL-1B and IL-6, recruit neutrophils to the affected area ( 31 , 32 ). Elevated serum IL-6 levels in severe TBI patients have been found to correlate with poorer outcomes, suggesting intensified inflammation results in more prominent pathology ( 33 ). Within 24 hours of TBI, microglia release nitric oxide (NO), ROS, IL-1B, and IL-6, enhancing the recruitment and differentiation of monocytes into tissue macrophages ( 34 , 35 ). The nucleotide-binding oligomerization domain-like receptor pyrin domain-containing-3 (NLRP3) inflammasome activation by innate immune regulator, nuclear factor- κB (NFκB), in microglia and astrocytes contributes to a pro-inflammatory environment via caspase cleavage and IL-1B and IL-18 secretion ( 36 ). In TBI, microglial-secreted IL-6 and NFκB upregulate the aquaporin (AQP4) water channel in endothelia, which is known to promote brain edema ( 32 , 37 , 38 ). Chronic microglial activation following TBI has been posited as one of the drivers of long-term cognitive dysfunction seen in TBI, with one study of former National Football League players showing increased binding of the microglia-associated inflammatory marker, translocator protein (TSPO), in brain regions including the supramarginal gyrus and amygdala ( 39 ). In addition to the increase in inflammatory signaling in the brain parenchyma, the meninges is rapidly gaining interest as an area of immune activation in the context of TBI ( 40 , 41 ). Indeed, in recent neuroimaging studies, patients with mild TBI show meningeal abnormalities that may indicate areas of increased inflammation ( 42 ).
Lymphoid immune cells have also been demonstrated to have significant involvement in the inflammatory response to TBI. Following TBI, CD4+ T cells differentiate into T-regulatory (Treg) and T-helper (Th) subsets Th1, Th2, and Th17 ( 43 ). Th1 and Th17 play strong pro-inflammatory roles in the ensuing immune response. Th1 cells release IL-2, interferon-gamma (IFN-y), and tumor necrosis factor-alpha (TNF-a) to activate macrophages and increase BBB permeability ( 44 , 45 ). Human CD4+ Th17 cells, via IL-17 and IL-22 secretion, disrupt the BBB and cause CNS inflammation ( 46 ). CD4+ Th2 cells secrete neuroprotective cytokines IL-4 and IL-5, promoting anti-inflammatory processes ( 47 ). Th cell polarization is driven by microglial secretion of the chemokine CXCL10, promoting Th1 cell infiltration and overall brain inflammation ( 48 ). A previously understudied, but critically important player in this neuroinflammatory response to TBI is the immune cell-rich lymphoid tissue found in the skull.
Immune contribution of the skull
Although the skull has been largely overlooked in neurotrauma research, it contains millions of immune cells in a proximal location to the brain and is thus poised to act as a significant modulator of CNS function via regulation of skull-meningeal trafficking ( Figure 1 ). The immune compartment in the diploe of the skull calvarium may also play a pivotal role in the immune activation following TBI via its direct access to arachnoid CSF absorption sites in the meninges through short ossified paravascular channels ( 49 , 50 ). Differentiated from other bone marrow compartments by its distinct transcriptomic and proteomic profile, calvarium bone marrow has been identified as a major source of immune cell recruitment to the CNS following injury to the brain ( 51 ). Multiple studies have demonstrated infiltration of skull-derived immune cells into the CNS following brain injury, indicating a previously uninvestigated role of skull bone marrow in contributing to the inflammatory response to TBI ( 52 ). The bone marrow is a complex environment containing various cell types of endothelial cells, osteocytes, and matrix components that support the immune system and hematopoietic stem cells (HSCs) ( 53 – 55 ). Within this environment, HSC proliferation and differentiation play a pivotal role in immune responses, giving rise to both lymphoid and myeloid cell lineages ( 56 , 57 ). In fact, during the acute inflammatory response to TBI, there is a well-characterized shift in HSC differentiation towards the myeloid cell lineage in the nearby skull marrow ( 58 ). Furthermore, damage to the skull has been shown to directly influence immune dynamics in the brain, with murine models of a weight-drop TBI demonstrating increased inflammation-related gene expression of TNF-α and TIMP-1 in the brain tissue of mice with skull fractures compared to those without ( 59 ). Mice with skull fractures also had significantly worse Neurological Severity Scores (NSS) than those without, and skull fracture severity correlated positively with NSS ( 59 ). It is not clear whether TBI-associated with or even without skull fractures resulted in greater leukocyte infiltration into the brain. Notably, murine models of CNS trauma demonstrate the presence of myeloid progenitors in the meninges during neuroinflammation, which are otherwise absent without trauma ( 60 , 61 ). Combined with findings that indicate myeloid cell migration from both the skull and peripheral blood, evidence strongly suggests a role for skull bone marrow in the initial immune response to TBI ( 17 , 62 ).
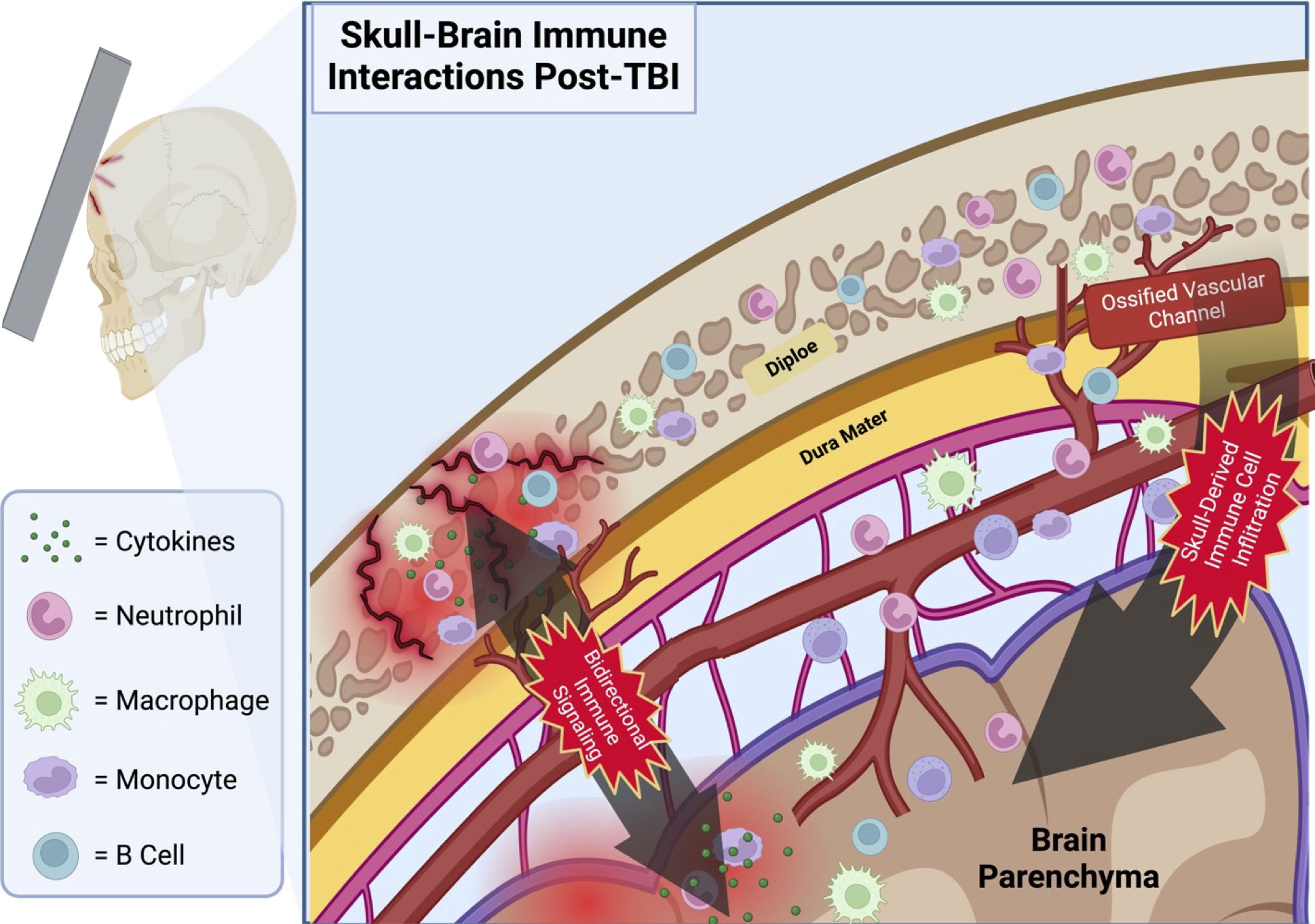
Figure 1 Proposed schematic depicting the infiltration of skull-derived immune cells into the brain parenchyma via ossified vascular channels following TBI with microfractures. Immune cells located in the calvarial bone marrow of the skull’s diploe have a direct portal to the meninges via the shared vasculature penetrating this cancellous bone tissue. Note the bidirectional travel of immune signaling molecules through these same channels. Illustration created with BioRender.com .
One of the main vehicles proposed to enable the transmigration of immune cells into the brain parenchyma CSF has also been implicated in signaling myeloid cell movement from the skull BM to the meninges by accessing skull diploe and mobilizing immune cells following insult ( 63 ). Additionally, these vascular portals between the CNS and the skull enable traffic in both directions, with immune factors from the CNS traveling to bone marrow niches in the skull to drive HSC differentiation and recruitment ( 64 ). The greatest source of this heterogeneity arises from differing genetic profiles of the resident myeloid and B cells. Multiple studies have demonstrated the presence of calvaria-derived neutrophils, monocytes, macrophages, and B cells in the meninges and brain parenchyma ( 17 ). While the precise role of the skull-derived hematopoietic cells in TBI remains uncertain, the existing literature indicates a clear mechanism for immune cell infiltration from the skull’s BM into the brain parenchyma following injury.
Importantly, the skull-meninges interface described here is bidirectional. This is supported by studies showing that CSF can exit into skull bone marrow to instruct hematopoietic response to neuroinflammatory conditions, including infection, stroke, and multiple sclerosis ( 64 , 65 ).Additionally, this skull-meninges connection may not only be a route of traffic for immune cells, but also secretory factors which may further modulate neuroinflammation following TBI. Unfortunately, there is to date little research directly investigating the changes to calvaria bone marrow composition following TBI. While there is no longer doubt as to the neuroinflammatory contribution of skull-derived immune cells located in the dura following brain injury, whether the neuroimmune response is preferential to migration from local bone marrow sites over circulating, bloodborne immune cells is a question which requires further investigation ( 17 ).
Glymphatic-meningeal lymphatic circulation
An important consideration to account for in the discussion of CSF circulatory dynamics between the skull and the meninges is TBI’s impact on paravascular glymphatic and perivascular lymphatic function in the meninges and brain. Normally responsible for the clearance of neurotoxic substances such as DAMPs, Aβ aggregates, tau proteins, and alpha-synuclein aggregates, the glymphatic and lymphatic systems in the brain play a vital role in recovery from TBI and are a burgeoning area of research in the field ( 66 ). TBI has been shown to significantly impact the rate of flow in these drainage systems. Following injury, the rate of paravascular drainage is slowed by as much as 60%, causing a subsequent reduction in the movement of interstitial fluid through the brain and an increase in protein accumulation in the parenchyma ( 67 ). Furthermore, it has recently been shown that enhancement of glymphatic-lymphatic drainage via a “nano-plumber” technology results in a significant improvement in neurological function in rodents following TBI ( 68 ). This treatment works by returning injured tissue to a homeostatic microenvironment via dampening the local microglial response and recovering healthy vascular and glymphatic-meningeal lymphatic circulation to support the clearance of noxious material and pro-inflammatory immune cells. The disruption to paravascular drainage in the parenchyma may well extend to the meningeal-skull marrow paravascular interface following TBI, however, the consequence of such a disruption for the function of immune signaling and migration between the skull and meninges is currently unknown.
Skull fracturing and morphological adaptation to TBI
Despite the dissipative effect on impact energy that may occur when the skull fractures in response to mechanical trauma, one of the greatest clinical risk factors for mortality and worsened outcomes in severe TBI is the presence of fractures to the skull vault or skull base ( 69 ). In the weight-drop animal model of TBI, skull fractures are reported to induce a significant increase in the inflammatory response compared to TBI mice without fractures ( 59 ). Due to the heterogeneity of cranial bone thickness and geometry, the likelihood of fracture varies significantly based on which region of the skull an impact occurs. Microfracturing is rarely examined in imaging studies but may be a key predictor of more serious fracturing with repeated head impacts. Recent studies investigating impact force dynamics on the human skull suggest that the temporal region is the area most susceptible to fracture, largely due to its thinner diploe compared to more resilient areas of the skull in the frontal, parietal, and occipital regions ( 19 ). The skull also appears to exhibit mechanosensitive adaptations to physical impact, increasing in thickness in the area exposed to the impact force in a dose-dependent manner. Preliminary research shows that this bone anabolic effect may be mediated in part by the cannabinoid-1 receptor ( 70 ). Furthermore, the underlying meninges appears to respond in a similarly localized fashion, mounting a dynamic transcriptomic response that is exacerbated with age ( 21 ). As with the potential role of the skull’s immune response in modulating TBI outcome, the influence of changes to skull morphology at both the macroscopic and cellular levels following TBI may serve as another path to explore potential therapeutic interventions for recovery from TBI.
Experimental models for TBI
Due to the heterogeneity of injuries associated with TBI, there are a number of different experimental animal models to mimic different aspects of both primary and secondary TBI progression and pathology. The current prevailing models in the TBI literature include controlled cortical impact (CCI), weight drop-impact acceleration injury, fluid percussion injury, blast injury, and penetrating ballistic-like brain injury ( 71 ). Although each of these models comes with its own advantages and disadvantages, experimental paradigms such as the weight drop-impact acceleration model and other closed-head models of TBI are better suited to study the skull-brain axis of immunity due to their comparatively less invasive manipulation of the calvarium. However, evidence of leukocyte infiltration is absent in most weight drop models of mild concussion. Injury models, such as CCI, that involve direct manipulation of the skull tissue prior to injury induction, most often in the form of a partial craniotomy over the impact location, may serve to obscure the potential influence of proximal skull tissue on injury progression and recovery following TBI. This is evidenced clinically by the high proportion of patients who undergo craniotomy experiencing fever in the post-operative period ( 72 ). Furthermore, as CT analysis of skull fracturing is commonly used for clinical diagnosis of TBI ( 73 ), models that incorporate this aspect of TBI pathology will be especially useful in future research investigating the influence of the skull in TBI secondary injury and recovery.
As one of the leading causes of neurological disability in the world, the study of TBI pathophysiology and its potential therapeutic interventions is a matter of pressing importance. Despite this, investigation of nearby tissues with preferential access and influence over immune dynamics within the brain is an area of relative neglect in TBI literature until recently. With the discovery of vascular channels by which the immune compartments of the brain and skull may communicate, there is a greater impetus than ever before to expand research seeking to investigate the role of this connection and how it may be incorporated into future treatment approaches.
Author contributions
GG: Writing – original draft. PD: Writing – original draft. BW: Writing – original draft. RR: Conceptualization, Funding acquisition, Supervision, Writing – review & editing.
The author(s) declare financial support was received for the research, authorship, and/or publication of this article. This work was supported by a grant from the NIH R00NS116032 (to RR).
Conflict of interest
The authors declare that the research was conducted in the absence of any commercial or financial relationships that could be construed as a potential conflict of interest.
Publisher’s note
All claims expressed in this article are solely those of the authors and do not necessarily represent those of their affiliated organizations, or those of the publisher, the editors and the reviewers. Any product that may be evaluated in this article, or claim that may be made by its manufacturer, is not guaranteed or endorsed by the publisher.
1. Siebold L, Obenaus A, Goyal R. Criteria to define mild, moderate, and severe traumatic brain injury in the mouse controlled cortical impact model. Exp Neurol . (2018) 310:48–57. doi: 10.1016/j.expneurol.2018.07.004
PubMed Abstract | CrossRef Full Text | Google Scholar
2. Tsai YC, Rau CS, Huang JF, Chang YM, Chia KJ, Hsieh TM, et al. The association between skull bone fractures and the mortality outcomes of patients with traumatic brain injury. Emerg Med Int . (2022) 2022:1296590. doi: 10.1155/2022/1296590
3. Heegaard WG, Biros M. Skull Fractures in Adults (2016). Available online at: https://wwwuptodatecom/contents/skull-fractures-in-adults .
Google Scholar
4. Zaloshnja E, Miller T, Langlois JA, Selassie AW. Prevalence of long-term disability from traumatic brain injury in the civilian population of the United States, 2005. J Head Trauma Rehabil . (2008) 23:394–400. doi: 10.1097/01.HTR.0000341435.52004.ac
5. Selassie AW, Zaloshnja E, Langlois JA, Miller T, Jones P, Steiner C. Incidence of long-term disability following traumatic brain injury hospitalization, United States, 2003. J Head Trauma Rehabil . (2008) 23:123–31. doi: 10.1097/01.Htr.0000314531.30401.39
6. Bruns J Jr., Hauser WA. The epidemiology of traumatic brain injury: A review. Epilepsia . (2003) 44:2–10. doi: 10.1046/j.1528-1157.44.s10.3.x
CrossRef Full Text | Google Scholar
7. National Academies of Sciences E, Medicine, Health, Medicine D, Board on Health Care S, Committee on the Review of the Department of Veterans Affairs Examinations for Traumatic Brain I. Evaluation of the Disability Determination Process for Traumatic Brain Injury in Veterans . Washington (DC: National Academies Press (2019).
8. Dash HH, Chavali S. Management of traumatic brain injury patients. Korean J Anesthesiol . (2018) 71:12–21. doi: 10.4097/kjae.2018.71.1.12
9. Robinson CP. Moderate and severe traumatic brain injury. Continuum (Minneap Minn) . (2021) 27:1278–300. doi: 10.1212/con.0000000000001036
10. Ladak AA, Enam SA, Ibrahim MT. A review of the molecular mechanisms of traumatic brain injury. World Neurosurg . (2019) 131:126–32. doi: 10.1016/j.wneu.2019.07.039
11. Galgano M, Toshkezi G, Qiu X, Russell T, Chin L, Zhao LR. Traumatic brain injury: current treatment strategies and future endeavors. Cell Transplant . (2017) 26:1118–30. doi: 10.1177/0963689717714102
12. Payne WN, De Jesus O, Payne AN. Contrecoup Brain Injury. In: Statpearls . StatPearls Publishing, Treasure Island (FL (2023).
13. Drew LB, Drew WE. The contrecoup-coup phenomenon: A new understanding of the mechanism of closed head injury. Neurocrit Care . (2004) 1:385–90. doi: 10.1385/ncc:1:3:385
14. Mena JH, Sanchez AI, Rubiano AM, Peitzman AB, Sperry JL, Gutierrez MI, et al. Effect of the modified glasgow coma scale score criteria for mild traumatic brain injury on mortality prediction: comparing classic and modified glasgow coma scale score model scores of 13. J Trauma . (2011) 71:1185–92. doi: 10.1097/TA.0b013e31823321f8
15. Haydel MJ. Evaluation of Traumatic Brain Injury, Acute . BMJ Best Practice (2023). Available at: https://bestpractice.bmj.com/topics/en-us/515 .
16. Yellinek S, Cohen A, Merkin V, Shelef I, Benifla M. Clinical significance of skull base fracture in patients after traumatic brain injury. J Clin Neurosci . (2016) 25:111–5. doi: 10.1016/j.jocn.2015.10.012
17. Cugurra A, Mamuladze T, Rustenhoven J, Dykstra T, Beroshvili G, Greenberg ZJ, et al. Skull and vertebral bone marrow are myeloid cell reservoirs for the meninges and cns parenchyma. Science . (2021) 373. doi: 10.1126/science.abf7844
18. Mills WA 3rd, Coburn MA, Eyo UB. The emergence of the calvarial hematopoietic niche in health and disease. Immunol Rev . (2022) 311:26–38. doi: 10.1111/imr.13120
19. Zwirner J, Safavi S, Scholze M, Li KC, Waddell JN, Busse B, et al. Topographical mapping of the mechanical characteristics of the human neurocranium considering the role of individual layers. Sci Rep . (2021) 11:3721. doi: 10.1038/s41598-020-80548-y
20. Zwirner J, Ondruschka B, Scholze M, Workman J, Thambyah A, Hammer N. The dynamic impact behavior of the human neurocranium. Sci Rep . (2021) 11:11331. doi: 10.1038/s41598-021-90322-3
21. Semple BD, Panagiotopoulou O. Cranial bone changes induced by mild traumatic brain injuries: A neglected player in concussion outcomes? Neurotrauma Rep . (2023) 4:396–403. doi: 10.1089/neur.2023.0025
22. McAllister TW. Neurobiological consequences of traumatic brain injury. Dialogues Clin Neurosci . (2011) 13:287–300. doi: 10.31887/DCNS.2011.13.2/tmcallister
23. Kang JH, Ko YT. Intraosseous administration into the skull: potential blood-brain barrier bypassing route for brain drug delivery. Bioeng Transl Med . (2023) 8:e10424. doi: 10.1002/btm2.10424
24. Kolabas ZI, Kuemmerle LB, Perneczky R, Förstera B, Ulukaya S, Ali M, et al. Distinct molecular profiles of skull bone marrow in health and neurological disorders. Cell . (2023) 186:3706–25.e29. doi: 10.1016/j.cell.2023.07.009
25. Hall ED, Detloff MR, Johnson K, Kupina NC. Peroxynitrite-mediated protein nitration and lipid peroxidation in a mouse model of traumatic brain injury. J Neurotrauma . (2004) 21:9–20. doi: 10.1089/089771504772695904
26. Xiong Y, Gu Q, Peterson PL, Muizelaar JP, Lee CP. Mitochondrial dysfunction and calcium perturbation induced by traumatic brain injury. J Neurotrauma . (1997) 14:23–34. doi: 10.1089/neu.1997.14.23
27. Cheng G, Kong RH, Zhang LM, Zhang JN. Mitochondria in traumatic brain injury and mitochondrial-targeted multipotential therapeutic strategies. Br J Pharmacol . (2012) 167:699–719. doi: 10.1111/j.1476-5381.2012.02025.x
28. Balança B, Desmurs L, Grelier J, Perret-Liaudet A, Lukaszewicz AC. Damps and rage pathophysiology at the acute phase of brain injury: an overview. Int J Mol Sci . (2021) 22. doi: 10.3390/ijms22052439
29. Russo MV, McGavern DB. Inflammatory neuroprotection following traumatic brain injury. Science . (2016) 353:783–5. doi: 10.1126/science.aaf6260
30. Nizamutdinov D, Shapiro LA. Overview of traumatic brain injury: an immunological context. Brain Sci . (2017) 7. doi: 10.3390/brainsci7010011
31. Hazeldine J, Naumann DN, Toman E, Davies D, Bishop JRB, Su Z, et al. Prehospital immune responses and development of multiple organ dysfunction syndrome following traumatic injury: A prospective cohort study. PloS Med . (2017) 14:e1002338. doi: 10.1371/journal.pmed.1002338
32. Laird MD, Shields JS, Sukumari-Ramesh S, Kimbler DE, Fessler RD, Shakir B, et al. High mobility group box protein-1 promotes cerebral edema after traumatic brain injury via activation of toll-like receptor 4. Glia . (2014) 62:26–38. doi: 10.1002/glia.22581
33. Lustenberger T, Kern M, Relja B, Wutzler S, Störmann P, Marzi I. The effect of brain injury on the inflammatory response following severe trauma. Immunobiology . (2016) 221:427–31. doi: 10.1016/j.imbio.2015.11.011
34. Beschorner R, Nguyen TD, Gözalan F, Pedal I, Mattern R, Schluesener HJ, et al. Cd14 expression by activated parenchymal microglia/macrophages and infiltrating monocytes following human traumatic brain injury. Acta Neuropathol . (2002) 103:541–9. doi: 10.1007/s00401-001-0503-7
35. Colton CA. Heterogeneity of microglial activation in the innate immune response in the brain. J Neuroimmune Pharmacol . (2009) 4:399–418. doi: 10.1007/s11481-009-9164-4
36. O'Brien WT, Pham L, Symons GF, Monif M, Shultz SR, McDonald SJ. The nlrp3 inflammasome in traumatic brain injury: potential as a biomarker and therapeutic target. J Neuroinflamm . (2020) 17:104. doi: 10.1186/s12974-020-01778-5
37. Burda JE, Bernstein AM, Sofroniew MV. Astrocyte roles in traumatic brain injury. Exp Neurol . (2016) 275 Pt 3:305–15. doi: 10.1016/j.expneurol.2015.03.020
38. Jayakumar AR, Tong XY, Ruiz-Cordero R, Bregy A, Bethea JR, Bramlett HM, et al. Activation of nf-Kb mediates astrocyte swelling and brain edema in traumatic brain injury. J Neurotrauma . (2014) 31:1249–57. doi: 10.1089/neu.2013.3169
39. Coughlin JM, Wang Y, Munro CA, Ma S, Yue C, Chen S, et al. Neuroinflammation and brain atrophy in former nfl players: an in vivo multimodal imaging pilot study. Neurobiol Dis . (2015) 74:58–65. doi: 10.1016/j.nbd.2014.10.019
40. Bolte AC, Dutta AB, Hurt ME, Smirnov I, Kovacs MA, McKee CA, et al. Meningeal lymphatic dysfunction exacerbates traumatic brain injury pathogenesis. Nat Commun . (2020) 11:4524. doi: 10.1038/s41467-020-18113-4
41. Liu M, Huang J, Liu T, Yuan J, Lv C, Sha Z, et al. Exogenous interleukin 33 enhances the brain's lymphatic drainage and toxic protein clearance in acute traumatic brain injury mice. Acta Neuropathol Commun . (2023) 11:61. doi: 10.1186/s40478-023-01555-4
42. Russo MV, Latour LL, McGavern DB. Distinct myeloid cell subsets promote meningeal remodeling and vascular repair after mild traumatic brain injury. Nat Immunol . (2018) 19:442–52. doi: 10.1038/s41590-018-0086-2
43. Bouras M, Asehnoune K, Roquilly A. Immune modulation after traumatic brain injury. Front Med (Lausanne) . (2022) 9:995044. doi: 10.3389/fmed.2022.995044
44. Saravia J, Chapman NM, Chi H. Helper T cell differentiation. Cell Mol Immunol . (2019) 16:634–43. doi: 10.1038/s41423-019-0220-6
45. Raposo C, Graubardt N, Cohen M, Eitan C, London A, Berkutzki T, et al. Cns repair requires both effector and regulatory T cells with distinct temporal and spatial profiles. J Neurosci . (2014) 34:10141–55. doi: 10.1523/jneurosci.0076-14.2014
46. Kebir H, Kreymborg K, Ifergan I, Dodelet-Devillers A, Cayrol R, Bernard M, et al. Human th17 lymphocytes promote blood-brain barrier disruption and central nervous system inflammation. Nat Med . (2007) 13:1173–5. doi: 10.1038/nm1651
47. Xu L, Ye X, Wang Q, Xu B, Zhong J, Chen YY, et al. T-cell infiltration, contribution and regulation in the central nervous system post-traumatic injury. Cell Prolif . (2021) 54:e13092. doi: 10.1111/cpr.13092
48. Michlmayr D, McKimmie CS. Role of cxcl10 in central nervous system inflammation. Int J Interferon Cytokine Mediator Res . (2014) 6:1. doi: 10.2147/IJICMR.S35953
49. Herisson F, Frodermann V, Courties G, Rohde D, Sun Y, Vandoorne K, et al. Direct vascular channels connect skull bone marrow and the brain surface enabling myeloid cell migration. Nat Neurosci . (2018) 21:1209–17. doi: 10.1038/s41593-018-0213-2
50. Mazzitelli JA, Pulous FE, Smyth LCD, Kaya Z, Rustenhoven J, Moskowitz MA, et al. Skull bone marrow channels as immune gateways to the central nervous system. Nat Neurosci . (2023) 26:2052–62. doi: 10.1038/s41593-023-01487-1
51. Zeynep Ilgin K, Louis BK, Robert P, Benjamin F, Maren B, Ozum Sehnaz C, et al. Multi-omics and 3d-imaging reveal bone heterogeneity and unique calvaria cells in neuroinflammation. bioRxiv . (2021) 2021:12.24.473988. doi: 10.1101/2021.12.24.473988
52. Shi SX, Shi K, Liu Q. Brain injury instructs bone marrow cellular lineage destination to reduce neuroinflammation. Sci Transl Med . (2021) 13. doi: 10.1126/scitranslmed.abc7029
53. Calvi LM, Link DC. Cellular complexity of the bone marrow hematopoietic stem cell niche. Calcif Tissue Int . (2014) 94:112–24. doi: 10.1007/s00223-013-9805-8
54. Schroeder T. Hematopoietic stem cell heterogeneity: subtypes, not unpredictable behavior. Cell Stem Cell . (2010) 6:203–7. doi: 10.1016/j.stem.2010.02.006
55. Cheng W, Ge Q, Wan L, Wang X, Chen X, Wu X. A method to establish a mouse model of bone marrow microenvironment injury. Exp Anim . (2017) 66:329–36. doi: 10.1538/expanim.16-0116
56. Feuerer M, Beckhove P, Garbi N, Mahnke Y, Limmer A, Hommel M, et al. Bone marrow as a priming site for T-cell responses to blood-borne antigen. Nat Med . (2003) 9:1151–7. doi: 10.1038/nm914
57. Parretta E, Cassese G, Barba P, Santoni A, Guardiola J, Di Rosa F. Cd8 cell division maintaining cytotoxic memory occurs predominantly in the bone marrow. J Immunol . (2005) 174:7654–64. doi: 10.4049/jimmunol.174.12.7654
58. Baleviciute A, Keane L. A skull bone marrow niche for antitumour neutrophils in glioblastoma. Nat Rev Immunol . (2023) 23:414. doi: 10.1038/s41577-023-00895-5
59. Zvejniece L, Stelfa G, Vavers E, Kupats E, Kuka J, Svalbe B, et al. Skull fractures induce neuroinflammation and worsen outcomes after closed head injury in mice. J Neurotrauma . (2020) 37:295–304. doi: 10.1089/neu.2019.6524
60. Koeniger T, Bell L, Mifka A, Enders M, Hautmann V, Mekala SR, et al. Bone marrow-derived myeloid progenitors in the leptomeninges of adult mice. Stem Cells . (2021) 39:227–39. doi: 10.1002/stem.3311
61. Cai R, Pan C, Ghasemigharagoz A, Todorov MI, Förstera B, Zhao S, et al. Panoptic imaging of transparent mice reveals whole-body neuronal projections and skull-meninges connections. Nat Neurosci . (2019) 22:317–27. doi: 10.1038/s41593-018-0301-3
62. Spijkerman R, Hesselink L, Bongers S, van Wessem KJP, Vrisekoop N, Hietbrink F, et al. Point-of-care analysis of neutrophil phenotypes: A first step toward immuno-based precision medicine in the trauma icu. Crit Care Explor . (2020) 2:e0158. doi: 10.1097/cce.0000000000000158
63. Mazzitelli JA, Smyth LCD, Cross KA, Dykstra T, Sun J, Du S, et al. Cerebrospinal fluid regulates skull bone marrow niches via direct access through dural channels. Nat Neurosci . (2022) 25:555–60. doi: 10.1038/s41593-022-01029-1
64. Pulous FE, Cruz-Hernández JC, Yang C, Kaya Z, Paccalet A, Wojtkiewicz G, et al. Cerebrospinal fluid can exit into the skull bone marrow and instruct cranial hematopoiesis in mice with bacterial meningitis. Nat Neurosci . (2022) 25:567–76. doi: 10.1038/s41593-022-01060-2
65. Ren H, Liu Q. Skull and vertebral bone marrow in central nervous system inflammation. Fundam Res . (2023) 4(2):246–50. doi: 10.1016/j.fmre.2023.01.012
66. Bacyinski A, Xu M, Wang W, Hu J. The paravascular pathway for brain waste clearance: current understanding, significance and controversy. Front Neuroanat . (2017) 11:101. doi: 10.3389/fnana.2017.00101
67. Bolte AC, Lukens JR. Neuroimmune cleanup crews in brain injury. Trends Immunol . (2021) 42:480–94. doi: 10.1016/j.it.2021.04.003
68. Tong S, Xie L, Xie X, Xu J, You Y, Sun Y, et al. Nano-plumber reshapes glymphatic-lymphatic system to sustain microenvironment homeostasis and improve long-term prognosis after traumatic brain injury. Adv Sci (Weinh) . (2023) 10:e2304284. doi: 10.1002/advs.202304284
69. Fujiwara G, Okada Y, Ishii W, Iizuka R, Murakami M, Sakakibara T, et al. Association of skull fracture with in-hospital mortality in severe traumatic brain injury patients. Am J Emerg Med . (2021) 46:78–83. doi: 10.1016/j.ajem.2021.03.020
70. Eger M, Bader M, Bree D, Hadar R, Nemirovski A, Tam J, et al. Bone anabolic response in the calvaria following mild traumatic brain injury is mediated by the cannabinoid-1 receptor. Sci Rep . (2019) 9:16196. doi: 10.1038/s41598-019-51720-w
71. Xiong Y, Mahmood A, Chopp M. Animal models of traumatic brain injury. Nat Rev Neurosci . (2013) 14:128–42. doi: 10.1038/nrn3407
72. Huang JH, Wang TJ, Wu SF, Liu CY, Fan JY. Post-craniotomy fever and its associated factors in patients with traumatic brain injury. Nurs Crit Care . (2022) 27:483–92. doi: 10.1111/nicc.12640
73. Borg J, Holm L, Cassidy JD, Peloso PM, Carroll LJ, von Holst H, et al. Diagnostic procedures in mild traumatic brain injury: results of the who collaborating centre task force on mild traumatic brain injury. J Rehabil Med . (2004) 43 Suppl):61–75. doi: 10.1080/16501960410023822
Keywords: TBI - traumatic brain injury, bone marrow, skull & brain, neuroinflammation, Myelopoiesis
Citation: Goodman GW, Devlin P, West BE and Ritzel RM (2024) The emerging importance of skull-brain interactions in traumatic brain injury. Front. Immunol. 15:1353513. doi: 10.3389/fimmu.2024.1353513
Received: 10 December 2023; Accepted: 01 April 2024; Published: 11 April 2024.
Reviewed by:
Copyright © 2024 Goodman, Devlin, West and Ritzel. This is an open-access article distributed under the terms of the Creative Commons Attribution License (CC BY) . The use, distribution or reproduction in other forums is permitted, provided the original author(s) and the copyright owner(s) are credited and that the original publication in this journal is cited, in accordance with accepted academic practice. No use, distribution or reproduction is permitted which does not comply with these terms.
*Correspondence: Rodney M. Ritzel, [email protected]
Disclaimer: All claims expressed in this article are solely those of the authors and do not necessarily represent those of their affiliated organizations, or those of the publisher, the editors and the reviewers. Any product that may be evaluated in this article or claim that may be made by its manufacturer is not guaranteed or endorsed by the publisher.
- Search Menu
- Advance articles
- Author Guidelines
- Submission Site
- Open Access
- About Cerebral Cortex
- Editorial Board
- Advertising and Corporate Services
- Journals Career Network
- Self-Archiving Policy
- Dispatch Dates
- Terms and Conditions
- Journals on Oxford Academic
- Books on Oxford Academic

- < Previous
Olfactory function after mild traumatic brain injury in children—a longitudinal case control study

- Article contents
- Figures & tables
- Supplementary Data
Janine Gellrich, Claudia Zickmüller, Theresa Thieme, Christian Karpinski, Guido Fitze, Martin Smitka, Maja von der Hagen, Valentin A Schriever, Olfactory function after mild traumatic brain injury in children—a longitudinal case control study, Cerebral Cortex , Volume 34, Issue 4, April 2024, bhae162, https://doi.org/10.1093/cercor/bhae162
- Permissions Icon Permissions
The prevalence of posttraumatic olfactory dysfunction in children after mild traumatic brain injury ranges from 3 to 58%, with potential factors influencing this variation, including traumatic brain injury severity and assessment methods. This prospective longitudinal study examines the association between mild traumatic brain injury and olfactory dysfunction in children. Seventy-five pediatric patients with mild traumatic brain injury and an age-matched healthy control group were enrolled. Olfactory function was assessed using the Sniffin’ Sticks battery, which focuses on olfactory threshold and odor identification. The study found that children with mild traumatic brain injury had impaired olfactory function compared with healthy controls, particularly in olfactory threshold scores. The prevalence of olfactory dysfunction in the patient group was 33% and persisted for 1 yr. No significant association was found between traumatic brain injury symptoms (e.g. amnesia, loss of consciousness) and olfactory dysfunction. The study highlights the importance of assessing olfactory function in children after mild traumatic brain injury, given its potential impact on daily life. Although most olfactory dysfunction appears transient, long-term follow-up is essential to fully understand the recovery process. The findings add valuable insights to the limited literature on this topic and urge the inclusion of olfactory assessments in the management of pediatric mild traumatic brain injury.
Email alerts
Citing articles via.
- Recommend to your Library
Affiliations
- Online ISSN 1460-2199
- Copyright © 2024 Oxford University Press
- About Oxford Academic
- Publish journals with us
- University press partners
- What we publish
- New features
- Open access
- Institutional account management
- Rights and permissions
- Get help with access
- Accessibility
- Advertising
- Media enquiries
- Oxford University Press
- Oxford Languages
- University of Oxford
Oxford University Press is a department of the University of Oxford. It furthers the University's objective of excellence in research, scholarship, and education by publishing worldwide
- Copyright © 2024 Oxford University Press
- Cookie settings
- Cookie policy
- Privacy policy
- Legal notice
This Feature Is Available To Subscribers Only
Sign In or Create an Account
This PDF is available to Subscribers Only
For full access to this pdf, sign in to an existing account, or purchase an annual subscription.
The Busted Melon: A Case of Traumatic Brain Injury
Traumatic brain injury is the leading cause of death and disability in children and young adults in the United States. It is also a major concern among people over 75, with high rates of death and hospitalization. 1 Common mechanisms of injury over all age groups include motor vehicle crashes, falls, injuries from firearms, and assaults. It has been a prevailing thought that there is little that prehospital care providers can do for patients with traumatic brain injuries, but recent studies and improved protocols reveal that EMS has a considerable impact on positive patient outcomes.
Case Introduction
EMS responds to a 28-year-old male who fell off his bicycle, and was not wearing a helmet. A passer-by found the cyclist semi-conscious, lying on the road for an unknown period. He assisted the cyclist to his feet and then sat him on the front seat of his car. Initial assessment reveals: Airway – patent with large amounts of vomit observed on the patient’s chin, mouth, shirt and on the ground by his feet; C Spine – potentially compromised, due to a suspected traumatic mechanism of injury; Breathing – regular rhythm and depth, slightly labored; Pulses present in all four extremities. The patient is now experiencing periods of lucidity, alternating with response only to voice.
When awake, the patient states he has no allergies, took his insulin and Lipitor today, and has a history of diabetes and high cholesterol. He states he was riding for an hour, gradually becoming dizzy and weak, and then thinks he passed out and crashed. The patient says he woke up laying on the road with someone hovering above him, asking if he was okay. There is no damage noted to the bike suggesting a collision with a vehicle. Vitals: pulse 98, ventilations 24, SpO 2 98%, BP 138/90, BGL 45 mg/dL, GCS 13 (E3, V4, M6).
Physical exam reveals a large hematoma on the right parietal/temporal area and crepitus when the cranium is palpated; pupils are equal but react sluggishly; abrasions on the right side of his face, no drainage observed from the nose or ears; posterior neck painful when palpated (no deformity), no jugular venous distention observed; abrasions on lateral right chest and arm; lung sounds are clear throughout, no palpated chest deformity; abdomen is soft and non-distended; pelvis is intact with slight pain upon palpation of right iliac crest; abrasions observed on the right lateral thigh and leg; distal pulses present – movement and sensation intact in all four extremities. Exam of the posterior trunk and legs is unremarkable except for abrasions. A cervical collar is applied and a scoop stretcher is carefully placed under the patient. He is then moved onto the ambulance cot for transport.
Primary and Secondary Traumatic Brain Injury (TBI)
There are two distinct categories – primary and secondary TBI. Primary traumatic brain injury occurs during an initial impact when physical structures of the brain are displaced, often resulting in lacerated vessels, mechanical disruption of brain cells and increases in vascular permeability. 3 When the skull and brain are pierced, this is called a focal injury, and when an external force produces excessive movement of the brain within the skull, this is called a diffuse injury. Primary injury may be a skull fracture, epidural, subdural or intracerebral hematoma, coup and contrecoup contusions, concussion, impaled object, or penetrating injury from a bullet, knife or shrapnel.
Secondary traumatic brain injury furthers the cellular brain damage from the effects of a primary injury. This typically develops over a period of hours or days following the initial head trauma. Common mechanisms that lead to secondary brain injury are breakdown of the blood-brain barrier – causing cerebral edema, hypoxia, hypotension, hypercarbia, and increased intracranial pressure. Many of these secondary injuries may be preventable with early and effective airway management, ensuring good ventilation and oxygenation, and maintaining blood pressure.
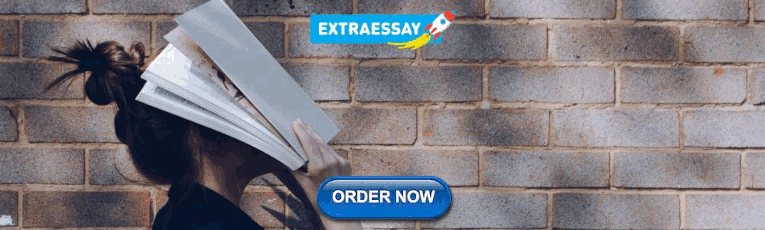
The Case Continues
The patient has a confirmed history of diabetes and has an altered LOC, so the EMS crew decides to administer dextrose to increase his serum glucose level. Cautious, due to the potential head injury, 12.5 grams is given IV. This increases the serum level to 106 mg/dL, but the patient suddenly begins to experience a tonic-clonic seizure. He begins forcefully vomiting and loses consciousness. The EMS crew carefully log rolls the patient onto his left side, while maintaining in-line positioning, and suctions his airway. Five milligrams of IV diazepam are administered, which stops the seizure. He still maintains a partially intact gag reflex, but his SpO 2 has decreased to 93%. A nasopharyngeal airway is inserted into the left nare, and oxygen is administered via non-rebreather mask flowing 15 Lpm. Repeat vitals: Ventilations 28; Pulse 106 regular with diminished pedal pulse strength, BP 146/88, BGL 104 mg/dL; SpO 2 98% with oxygen; GCS 10 (E2, V3, M5). The hematoma has not appeared to have grown in size, but the patient’s right pupil is larger when compared to the left and has become less reactive.
How does a TBI affect the brain?
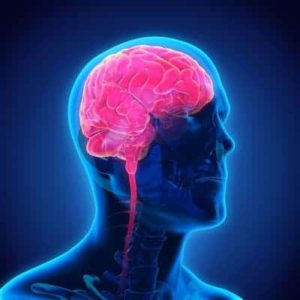
If one or more of the three cranial components decrease in volume from increased ICP, this also compromises cerebral perfusion pressure (CPP). Cerebral perfusion pressure is the pressure required to ensure blood flow to the brain. It is dependent upon intracranial pressure remaining constant as well as a sufficient mean arterial pressure (MAP). MAP is a measure of an average pressure exerted in the arteries during a single cardiac cycle – regulated by adjustments in cardiac output and systemic vascular resistance. Normal range for MAP is 70-110 mmHg, and normal range for ICP is 0-15 mmHg. Any change in either MAP or ICP will affect brain perfusion.
An adequate CPP is between 70-90 mmHg. When cerebral perfusion pressure drops below 70 mmHg for even short periods, cerebral hypoxia and ischemia can occur. The brain prevents a dangerous CPP drop via autoregulation . It is an automatic response that reacts to fluctuations in the systemic arterial pressure with an opposite reaction by the cerebral vessels. For example, an increase in systemic blood pressure causes the brain to constrict the cerebral arteries to limit cerebral blood volume and any increase in intracranial pressure. Conversely, if the patient’s systemic blood pressure decreases, the cerebral vessels dilate, allowing for increased blood flow and maintenance of brain perfusion. 3
Autoregulation is effective when the MAP is between 60-130 mmHg and when ICP is less than 40 mmHg. If the MAP rises above 130 mmHg, the cerebral vessels leak fluid into surrounding brain tissue and increase ICP. If the MAP drops below 60 mmHg, cerebral blood flow is insufficient and causes ischemic tissue injury. When ICP exceeds 40 mmHg, autoregulation is disrupted. The maintenance of cerebral blood flow now relies upon the brain being able to increase systemic blood pressure by peripheral vessel vasoconstriction. This does increase/maintain blood flow to the head, but forces a greater-than-normal volume of blood into the cranium. The cerebral vessels remain dilated due to autoregulation loss, resulting in a progressive increase in cerebral edema, intracranial pressure, and brain ischemia.
The patient is loaded into the ambulance for a 10-minute transport to the trauma center. Seizure activity does not return, and he appears postictal. In an attempt to assess mental status, the EMT applies a vigorous sternal rub, which causes the patient to moan loudly and flail his right arm away from midline. The EMT also observes the patient’s breathing progressively gets faster, then slows, stops, then repeats the cycle. The NRB mask is removed and the EMT begins to ventilate the patient with a BVM and oxygen. The patient does not resist the assisted ventilatory support and accepts insertion of an oropharyngeal airway. Repeat vitals: Pulse 80 regular, absent pedal pulses and diminished radial pulse strength; BP 162/70; Ventilations via BVM at 18/minute, SpO 2 99%; BGL 100 mg/dL; GCS 7 (E1, V2, M4). A repeat physical exam reveals no changes except the right pupil is further dilated and is not reactive to light. The patient is positioned supine on the ambulance cot and rapid transport is initiated.
Signs and Symptoms of Increased ICP and Herniation
The best indicator of increasing ICP is a changing mental status. 4 These changes can range from restlessness and confusion to irritability, combativeness and coma. Other indicators include severe headache, projectile vomiting, personality changes, slowing of speech, loss of reflexes, abnormal posturing, seizure activity, pupillary changes, abnormal breathing patterns, bradycardia and progressively increasing hypertension with an associated widening pulse pressure. These last three findings constitute the Cushing reflex, which is a primary sign of brainstem herniation.
As ICP increases, eventually the cranial vault completely fills. If ICP continues to increase further – continuing the expansion of contents, they are forced toward the only place in the skull that has a lower pressure, the foramen magnum. Anatomically, the brainstem is sandwiched between the expanding cerebrum, blood and CSF superiorly and the inferior foramen magnum. As ICP increase continues, the brainstem progressively gets compressed, and herniation begins.
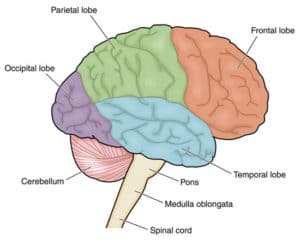
As ICP continues to increase, the midbrain is disabled and the pons now must try to compensate. Think of the pons as 2 pieces – a superior half and inferior half. The superior half is the home of the acceleration center. Overstimulation from ICP leads to the respiratory pattern acutely changing from Cheyne Stokes into central neurogenic hyperventilation. Once the superior pons is crushed and disabled, the inferior portion is overstimulated – home to the deceleration center. The patients’ breathing acutely becomes irregular in depth, rate and rhythm, Bradycardia worsens, pulse pressure continues to widen, and the patient’s blood pressure continues to rise. Systolic blood pressure can easily climb into the 200-250+ mmHg range. Unilateral or bilateral sluggish/inactive pupils may be observed, and painful stimulus produces an abnormal extensor response (decerebrate posturing).
When the midbrain and the pons’ functions are destroyed, the remaining active portion of the brainstem is the medulla oblongata. Its vegetative functions of maintaining respiration and circulation are rapidly compromised by the crushing force of the brain mass. Systemic blood pressure regulation is lost and the patient becomes profoundly hypotensive, resulting in the loss of cerebral perfusion. The patient’s breathing pattern becomes agonal, then apneic. Bradycardia decompensates into cardiac arrest in many cases. The pupils become fixed and dilated and there is neither an extensor nor a flexor response to painful stimulation. Such findings typically suggest a level of ICP that is not survivable.
Management Pearls
The prehospital management of increased ICP centers on maintaining and protecting the airway, breathing, and circulation in an attempt to control ICP and prevent a decrease in cerebral perfusion. A study published in JAMA Surgery: The Journal of the American Medical Association 5 highlights the importance of treating and preventing the three “H Bombs” (Hypoxia, Hypotension and Hyperventilation) that lead to irreversible brain damage and death following a brain injury. This study stems from a project known as EPIC: Excellence in Prehospital Injury Care.
Be sure to oxygenate any TBI patient to maintain an SpO 2 greater than 90% at all times, as even one episode of hypoxia can double the mortality of a head-injured patient. 6 Ideally, administer oxygen by the most appropriate method, to obtain an SpO 2 close to 100%. Pay particular attention when placing an advanced airway and the time it may take to do so. Prolonged and/or multiple airway insertion attempts may lead to rapid oxygen desaturations and increase the chance for hypoxia-related complications.
Hypotension
A single episode of hypotension is very detrimental to ischemic brain tissue and cerebral blood flow. Any episode of hypotension also doubles the mortality from head injuries. 6 If the patient is, or becomes hypotensive, infuse isotonic IV fluid to establish and maintain a systolic blood pressure of at least 90 mmHg. However, do not delay transport to establish an intravenous line in the field.
Hyperventilation
First responders have always been taught to hyperventilate patients with suspected TBI. However, recent research has shown that while hyperventilation lowers intracranial pressure in some instances, more often it deprives the brain of blood and oxygen due to the extreme reduction of serum carbon dioxide. The cerebral vasoconstriction that results causes the brain insult. Hyperventilation is not necessary with head-injury patients who do not have signs or symptoms of herniation, as their auto regulatory mechanism is still intact. EPIC recommends that TBI patients never be hyperventilated, even if herniation is suspected. It is a very rare event in the prehospital setting – what is observed as possible herniation, most times is not the case. When ventilating a patient suspected of having a TBI, the rate be should 10/minute for patients older than 15, 20/minute for patients 2 – 14 years old and 25/min for patients less than 2 years old. Each ventilation should be over one second and enough to initiate chest rise. Capnometry should be monitored throughout care and maintained between 35-45 mmHg. The EPIC results recommend the magic number be no higher than 40 mmHg. A normal capnograph waveform should be present as well. If not, assess for issues that may have altered the shape, angle or plateau of the waveform, as these sometimes contribute to patient decline.
Additionally, consider these points for a patient with suspected TBI. One, the severity of a traumatic brain injury, according to Glasgow Coma Score, is graded as: Mild 13-15, Moderate 9-12, and Severe 3-8. 2 The lower the score, the worse the prognosis and lessened ability for a patient to maintain their airway. Two, employ spinal motion restriction protocol, as warranted. Three, always consider rapid transport by the most-appropriate means to a trauma center capable of neurosurgery. Four, if a seizure occurs, administer a benzodiazepine to stop the seizure activity. Be careful with the dosage, however, so as not to induce hypotension. Post-seizure, check for reduced blood glucose levels and manage accordingly. Lastly, maintain a patient’s normal body temperature by the most appropriate method.
Case Conclusion
The paramedic decides to intubate the airway, due to the patient’s LOC being severely diminished and no gag reflex present. The tube is passed without difficulty or delay, secured, and the BVM is reattached along with in-line capnometry. Observing signs and symptoms of a traumatic brain injury and possible brainstem herniation, the EMT slows ventilations to 10/minute. He gives enough volume for observable chest rise, and maintains an EtCO 2 between 35-40 mmHg. Remaining transport time to the hospital is five minutes. Repeat vitals: Pulse 75 regular with a thready radial pulse; BP 178/62; SpO 2 99%; GCS 5 (E1, V1, M3). Another peripheral IV line is established, run at KVO rate and the cardiac monitor is applied – showing normal sinus rhythm. The hematoma appears to have increased in size and the right pupil is fixed and dilated. The remainder of the transport is unremarkable and full report is given to the receiving ED staff without incident. The patient is rushed to surgery where a large epidural bleed on the temporal area of the patient’s brain is evacuated and repaired.
- Traumatic Brain Injury in the United States Fact Sheet. Centers for Disease Control and Prevention, National Center for Injury Prevention and Control, Division of Unintentional Injury Prevention. January 12, 2015.
- Dawodu, S.T. (2019, June 27). Traumatic Brain Injury (TBI) – Definition, Epidemiology, Pathophysiology. Retrieved from: https://emedicine.medscape.com/article/326510-overview#a1
- Duncan, T., et.al. (2007, July 1) Beyond the Basics: Brain Injuries. Retrieved from: https://www.emsworld.com/article/10321801/beyond-basics-brain-injuries
- Snyder, S. (2012, February 6). Troubled Mind: The Lowdown on Increased ICP. Retrieved from: https://www.emsworld.com/article/10624283/troubled-mind-lowdown-increased-icp
- Bassett, J (2019, July 1). Retrieved from: https://www.emsworld.com/article/1222954/avoiding-three-h-bombs-prehospital-tbi-management
- Duckworth, R (2018, March 26) EPIC: An EMS-centered approach to head injuries. Retrieved from: https://www.ems1.com/ems-training/articles/378624048-EPIC-An-EMS-centered-approach-to-head-injuries/
About the Author
Chris Ebright is an Education Coordinator with the National EMS Academy, managing all aspects of initial paramedic education for Acadian Companies, Inc. in the Covington, Louisiana area. He has been a Nationally Registered paramedic for 24 years, providing primary EMS response along with land and air critical care transportation. Chris has educated hundreds of first responders, EMT’s, paramedics, and nurses for 23 years with his trademark whiteboard artistry sessions. Among his former graduates is the first native paramedic from the Cayman Islands. Chris’ passion for education is currently featured as a monthly article contributor, published on the Limmer Education website. He has been a featured presenter at numerous local, state and national EMS conferences over the past 12 years, and enjoys traveling annually throughout the United States meeting EMS professionals from all walks of life. Chris is a self-proclaimed sports, movie and rollercoaster junkie and holds a Bachelor of Education degree from the University of Toledo in Toledo, Ohio. He can be contacted via email at [email protected] or through his website www.christopherebright.com
Previous Post What You Need for AEMT/Paramedic Classes
Next post tablets, capsules, liquids: polypharmacy and the elderly, recommended for you.
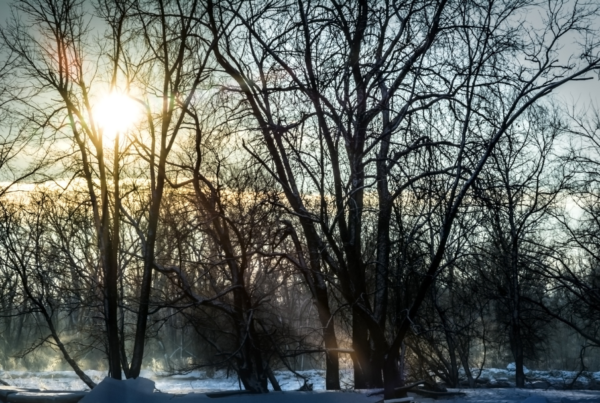
Sacred Cows Are Alive and Well in EMS
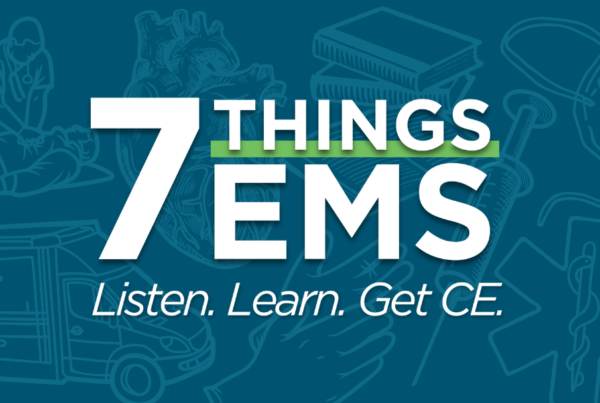
We use cookies to ensure best experience for you
We use cookies and other tracking technologies to improve your browsing experience on our site, show personalize content and targeted ads, analyze site traffic, and understand where our audience is coming from. You can also read our privacy policy , We use cookies to ensure the best experience for you on our website.
By choosing I accept, or by continuing being on the website, you consent to our use of Cookies and Terms & Conditions .
- Leaders Speak
- Study reveals novel therapeutic target for traumatic brain injury
Most traumatic brain injuries come as a result of falls, car crashes, or violent assaults, according to the Centers for Disease Control, but many also stem from sports accidents or certain military operations such as explosions. In each case, the external force is strong enough to move the brain within the skull, causing a significant breakdown in the blood-brain barrier and allowing blood to move in.

- Updated On Apr 20, 2024 at 06:40 AM IST
- Published On Apr 20, 2024 at 06:36 AM IST
All Comments
By commenting, you agree to the Prohibited Content Policy
Find this Comment Offensive?
- Foul Language
- Inciting hatred against a certain community
- Out of Context / Spam
Join the community of 2M+ industry professionals
Subscribe to our newsletter to get latest insights & analysis., download ethealthworld app.
- Get Realtime updates
- Save your favourite articles

- traumatic brain injury
- immunotherapy
- blood-brain barrier
- neurological diseases
- Gladstone Institutes
- Alzheimer's disease
- health news

An official website of the United States government
The .gov means it’s official. Federal government websites often end in .gov or .mil. Before sharing sensitive information, make sure you’re on a federal government site.
The site is secure. The https:// ensures that you are connecting to the official website and that any information you provide is encrypted and transmitted securely.
- Publications
- Account settings
Preview improvements coming to the PMC website in October 2024. Learn More or Try it out now .
- Advanced Search
- Journal List
- Med Sci Monit
- v.17(2); 2011

A case of “Borrowed Identity Syndrome” after severe traumatic brain injury
Maria pachalska.
1 Institute of Psychology, Gdansk University, Gdansk, Poland
2 Faculty of Psychology and Health Sciences, Frycz Modrzewski Cracow University, Cracow, Poland
Bruce Duncan MacQueen
3 Institute of English Literature, Gdansk University, Gdansk, Poland
Bozydar L. J. Kaczmarek
4 Institute of Psychology, Szczecin University, Szczecin, Poland
5 Institute of Psychology, Maria Curie-Sklodowska University, Lublin, Poland
Magdalena Wilk-Franczuk
6 Health and Medical Sciences Department, Frycz-Modrzewski Cracow University, Cracow, Poland
Izabela Herman-Sucharska
7 Magnetic Resonance Laboratory, Collegium Medicum, Jagiellonian University, Crakow, Poland
It is well known that traumatic brain injury often changes the way the patient perceives reality, which often means a distortion of the perception of self and the world. The purpose of this article is to understand the processes of identity change after traumatic brain injury.
Case Report
We describe progressive deterioration in personal identity in a former physician who had sustained a serious head injury (1998), resulting in focal injuries to the right frontal and temporal areas. He regained consciousness after 63 days in coma and 98 days of post-traumatic amnesia, but has since displayed a persistent loss of autobiographical memory, self-image, and emotional bonds to family and significant others. Qualitative ‘life-story’ interviewing was undertaken to explore the mental state of a patient whose subjective, “first person” identity has been disengaged, despite the retention of significant amounts of objective, “third person” information about himself and his personal history (though this was also lost at a later stage in the patient’s deterioration).
Identity change in our patient was characterized by a dynamic and convoluted process of contraction, expansion and tentative balance. Our patient tends to cling to the self of others, borrowing their identities at least for the period he is able to remember. Identity is closely connected with the processes of memory.
Conclusions
The results will be examined in relation to the microgenetic theory of brain function. The brain mechanisms that may account for these impairments are discussed. Findings from this study have important implications for the delivery of person-focused rehabilitation.
The traditional approach to disorders consequent to brain injury centers on the description of concrete symptoms that be shown to correlate significantly with injuries to particular brain regions. This approach began with the pioneering works of Broca [ 1 ] and Wernicke [ 2 ], and has continued in much contemporary work in neuropsychology [ 3 – 5 ]. A.R. Luria [ 6 ] tried to go beyond merely listing the particular symptoms observed, introducing the idea of the “basic defect,” i.e. a single underlying disorder that is presumably causing the manifold observable difficulties the patient manifests in performing the tasks involved in a neuropsychological examination. Thus a basic defect in phonemic hearing would affect performance in all tasks involved with that function, such as verbal memory, word repetition, reading, writing, etc., while performance should be unimpaired in tasks that do not require phonemic hearing. Only in this way would it be possible, in Luria’s view, to describe a syndrome characterized by a particular set of disorders following a specific brain lesion.
Luria’s approach was certainly a step forward, both in the better understanding of the nature of the symptoms observed in a particular patient and in conducting more effective therapy. Yet Luria’s approach was only able to explain some of the symptom changes observed in a patient during the process of recovery. It does not provide an explanation for the appearance of clinically distinct syndromes over the course of progressive deterioration, as observed in many post-TBI patients. One such patient will be described in the present report, with particular emphasis on some unusual disorders of personal identity in a former physician who sustained a serious brain injury due to a car accident in 1998. The injury resulted in focal injuries in the frontal and right temporal areas and coma lasting 63 days. The real purpose of this paper, however, is to explore the mind of a patient whose identity has been disrupted, and who has experienced the loss of his self image and relations with his immediate surroundings.
This case study follows patient PA from the age of 43 at the time of injury until his current age of 54 years. The patient is a board-certified gynecologist and obstetrician. Prior to the accident, he was the head of the OB-GYN department in a provincial hospital in southern Poland. He was married with three children, currently ranging in age from 13 to 26 years. The oldest daughter finished the Academy of Fine Arts in 2009 and is making a career as a painter. There were some problems with the marriage after his wife discovered that he had had a lover, who, as reported by his family and the lover herself, he had claimed to regard as the love of his life.
In November 1998, the car PA was driving struck a tree when he swerved to avoid a head-on collision. He incurred multi-organ injuries, including a very severe TBI. He remained conscious for a short period of time immediately after the accident, however, and his memory concerning the event itself seems to be intact as of this writing. An intracranial hematoma developed immediately after admission to hospital, and the patient lost consciousness. His Glasgow Coma Scale score 24 hours after the accident was 3, the lowest possible. He remained in a coma for 63 days, but was not operated; his post-injury amnesia lasted 98 days.
Neuroimaging ( Figure 1 ) shows damage mainly in the right hemisphere, most significantly affecting:

MRI scan of PA’s brain, performed four months post injury. ( A ) Axial SE T1 sequence: malacia in the right lower frontal gyrus (short arrow) and lower temporal gyrus (long arrow). ( B ) Axial FLAIR sequence: hyperintensive gliotic lesion, right lower frontal gyrus (arrow). ( C ) Axial FLAIR sequence: atrophy and malacia, right gyrus rectus (arrow).
- the gyrus rectus;
- the frontal lobe, especially the precentral orbital gyrus;
- the lower frontal gyrus;
- the lower, central and upper temporal gyrus.
When first examined by the authors in January 1999, the patient was found to be suffering from:
- hemiparesis;
- sleeping problems, including narcolepsy, occasional parasomnia and nightmares;
- mild post-traumatic aphasia, with naming problems;
- anosognosia;
- executive dysfunction;
- inability to perform activities of daily living (ADL);
- bouts of aggressiveness;
- visuospatial disorientation;
- identity disturbance (does not recognize himself, does not remember his name);
- autobiographical memory disturbance;
- prosopagnosia (confuses close relatives, does not recognize his children or extended family members).
Initial signs of misidentification were observed in February 1999. These included:
- inaccurate spatial orientation with confabulation, e.g. the clinic was identified as a garage and he was a mechanic;
- loss of the feeling of personal identity (the patient complained that he did not know who he was, despite the absence of objective symptoms of global amnesia);
- abrupt changes in personal habits and routines (including smoking and drinking);
- loss of personal history, social roles, and lifestyle.
At that time he was able to recognize (i.e. name) some personal items (e.g. keys, comb, toothbrush), but denied that they belonged to him. Later in his recovery, he was able to name objects relevant to his past, e.g. cigarettes, a bottle of cognac, but did not recognize these items as being connected with his previous experience.
We learned from an interview with his wife that on the day of the accident a major marital crisis had occurred, resulting from the patient’s infidelity. His wife had discovered that PA intended to go to a party with his lover. After a fierce quarrel he eventually backed down, and went with his wife instead; however, during the trip there was a sudden change in the situation on the road, and PA drove into a tree while passing a delivery truck. His wife did not suffer any serious injuries, because PA at the very last moment before the collision steered the car in such a way so as to take on himself the full force of the impact.
The patient’s mother is of the opinion, and has often repeated it, that the cause of the accident was the hysterical behavior of her daughter-in-law. The truth is that her son had always driven much too fast and recklessly; PA has loved sports cars since childhood, and dreamed of taking part in car rallies.
Very significant changes in his habits and behavior were noted in the 6 months following his arousal from coma. Prior to his accident he had been a vegetarian, a smoker, a moderate drinker, a known wit, who enjoyed social intercourse and parties. Following the injury, he had no recall of this lifestyle and personal history. At the same time PA exhibited symptoms of mirror sign, and a few months later of Capgras Syndrome, which included suspicion and confabulations regarding his wife and children (whom he called imposters), denial that he even knew his erstwhile lover, and even denial of his dog (who seemed not to recognize him when he returned home, and barked at him). However, facial recognition of public figures was preserved. Below are some examples of the patient’s problems:
Mirror sign
A physiotherapist named Jacek was helping PA stand in front of a full-length mirror:
Therapist (Th): Who is that, Peter? Who do you see there?
PA: I don’t know. Oh my God! That monster is staring at me [shouting].
Th: And who else do you see in the mirror?
PA: I don’t know, but maybe Jacek, I think you said so, isn’t that right?
Although he was not able to recognize himself in the mirror, he recognized and gave the name of the therapist. He also remembered his own name when asked. Ten minutes after this incident, however, he did not remember that he had been standing before a mirror or what the therapist’s name was.
Denial of the family
Another feature of the disorder was denial of the family. Here is an extract from an interview with the patient in the presence of his parents, his wife and his oldest daughter, who was 19 at the time:
Th: You are pleased that you’ve been visited by your family, aren’t you?
PA: Me? Of course not! I don’t have a family. I don’t know these people. My family was all killed in an accident.
Th: And…
PA: I don’t know these people. They are body doubles… doubles of my entire family or I don’t know [shouting]!
This seems a very clear case of Capgras syndrome [ 7 ]. Neuropsychologists and psychiatrists have known for nearly 100 years that a small number of psychiatric patients become profoundly suspicious of their closest relationships, often cutting themselves off from those who love them and care for them. They may insist that their spouse is an impostor, that their grown children are body doubles; that a caregiver, a close friend, even the entire family is fake, a duplicate version.
Denial of the lover
Another symptom we observed during this period was denial of the extra-marital relationship (supposedly “the love of his life”). Upon being visited by his former girlfriend in the hospital, he displayed complete non-recognition.
L: How are you feeling, darling? [attempts to kiss him]
PA: Don’t kiss me [shouting]!. I don’t know you!
After one hour the therapist asked him about the visit (which had been arranged by PA’s mother).
Th: So, you have been visited by your girlfriend?
PA: That hag is supposed to be my lover?
Th: She’s a beautiful woman, isn’t she?
PA: Perhaps I could consider that woman beautiful, yes… I would regard her as beautiful, she is about 40, isn’t she? No girlfriend of mine could be so old! Besides, I’ve never had a lover.
Although PA did not recognize his lover, a few minutes later in conversation he suddenly stated that it was not permissible for a physician to enter into such a close relationship with a patient – even though no one had yet said to him that the woman who was claiming to be his lover had previously been his patient, and in fact the subject of conversation had already changed. This might suggest that he recognized her as a former patient (which in point of fact is true), but not as his lover. On the other hand, his wife is also a doctor, so his “pangs of conscience” might be related to his marriage, though that seems rather far-fetched. It is nearly impossible to establish, in his cognitive state, to what extent his failure to acknowledge his lover is repressed guilt, and to what extent it should be called amnesia, or confusion.
About half an hour after his lover had left the room, the patient asked the therapist, “Aren’t you… hmm… my fiancée or something… she must be somewhere, but I don’t know where she is and nobody can find her.”
It is possible that he was remembering the unpleasant conversation with his fiancée and was somehow looking for her to make things right. Some traces may have been maintained In his memory, then, of his feelings for this woman, and these memories were activated, with a marked delay, by the visit of his girlfriend. In his state of cognitive confusion he misidentified his therapist as his lover, but the emotional memory indicating that the lover had been lost was essentially correct.
Denial of the dog
One of the most interesting aspects of PA’s problems with autobiographical memory is his complete denial of having ever had a dog, when before the accident he and the dog had been extremely close. This constitutes a first in reported cases of a similar nature.
T: Is this your dog? [in the presence of his pet, a dachshund, who is barking at him. Apparently, the dog does not exhibit positive feelings towards the patient here and now].
PA: Nothing of the kind! A lump of fur like that! I don’t own a dog. I wouldn’t want such a rubbishy thing! I’m afraid of this dog. It wants to bite me!
PA was able to recognize public figures, but during this period he was watching an excessive amount of television, and had become interested in politics only after the accident. It is quite possible, however, that these faces of public figures constitute islands of episodic or autobiographical memory, which excludes any assumption that his disturbances might be linked to difficulties in facial recognition per se . After being shown pictures of two important Polish politicians he stated:
“… here is the President and the Prime Minister… they have power… It’s them who changed the calendar… and everyone thinks that I’m 40 when I’m really 20…”.
Identity misidentification
This last utterance showed another important characteristic of PA’s mental state, namely, the problems with identity. Not only was he unable to state his age, but he reacted with anger to an attempt by the therapist to specify his true identity, which is illustrated by the following conversation:
Th: Who are you?
PA: Who am I? I don’t know! Perhaps you could tell me?
Th: You are a doctor – a gynecologist.
PA: No! I’m too young to be a doctor.
Th: How old are you?
PA: Probably 7 or 8 years old.
It was eventually established that he possessed some murky recollections of childhood up to the age of eight. At that time he had dreamed of becoming a car mechanic, and now he sometimes claimed to be one. He also vaguely remembered his first year at school, but from the age of eight. He complained that he felt lost. This can be noted in his commentary accompanying his drawing of a ladybug ( Figure 2 ):

Patient’s drawing representing himself as a ladybug. The patient’s inscription reads as follows: “My insides remind me of a ladybug. She’s looking for something, because it’s empty inside her, like it’s empty inside me.”
“My inner self reminds me of a ladybug. It’s obviously looking for something because she feels foggy and empty… LIKE ME… everything has to be searched for ”.
The loss of identity was accompanied by delusions, which is illustrated by the following utterance:
… the government has not only changed the money and I can’t recognize it, but also the calendar to avoid paying the life annuity… they added 30 years to the established calendar and as a result I am supposed to be 45 years old, but really, I’m 25. They want to get rid of me. I’m scared.
He would often change his stated age depending on the person with whom he found himself in contact.
Over time PA began to develop an “auto-Fregoli” syndrome. His lack of a personal sense of identity made him borrow the identity of others. Below are presented examples of some of the more interesting identities assumed by PA:
- When he was on the ward with a patient named Andrzej, who had global amnesia and maintained that he was a six- year-old clone, PA began shouting that the doctors had cloned him, too, and that now he was a six-year-old monster.
- When he was on the ward with a 19-year-old patient named Jurek, who had had a knee operation, he shouted that he couldn’t walk because he had had a knee operation and would have to use a wheelchair; he also maintained that his name was Jurek.
- When PA met Zbyszek, a 29-year-old art therapist, he took the brushes from him and refused to give them back. He shouted that he had to paint a picture in the open because he had to earn a living. He answered to the name Zbyszek and claimed to be 29 years old.
- When a 20-year-old hairdresser was cutting his hair, he took her scissors from her and refused to give them back. He shouted that she could not take away his means of livelihood.
- When the young chaplain of the physiotherapy department, whom the patient had taken a liking to, was distributing communion on the ward, PA took his Bible, which had been left for a moment on the table, and refused to give it back. He shouted that they wanted to steal the Bible from him and that he would have nothing to pray from.
All these temporary acquired identities were based on situational stimuli: the identities borrowed by this patient are content-specific. It is also important to state that these identities have a temporal frame: for acquired identities, approximately 1–2 hours, which seems to be a factor of the maximum duration of his episodic memory. He would momentarily associate himself with an object or person which in some way attracted his attention, though what motivation there was for this particular object or person remains unknown, when other “opportunities” were ignored. The individuals selected were never older than their late 20s, and not infrequently they were children.
Of importance is the fact that after an hour (or sometimes two) PA would forget about his newly adopted identity, and again did not really know who he was. Sometimes he accepted his true identity, as suggested by a researcher. However, in general he would protest, and present himself as a car mechanic.
PA’s identity disturbances are persistent. The patient still does not know who he is. He continues to ascribe to himself the identity of those with whom he comes into contact. He believes that his family all died in an accident, but does not show any emotional reaction to this. He complains that he loves no one and no one loves him. He is totally alone, but he has no clear recognition of his situation. After reading about Cotard’s delusion, a rare psychiatric syndrome in which people consider themselves to be dead, he consequently claimed that he was just such a case and was in fact dead, or perhaps reincarnated [ 8 ].
It came as a surprise, however, to discover, 3 years after the accident, that his medical knowledge was very well preserved. After meeting a TBI patient who had been pregnant and had lost her child, he advised her in a very professional way, and told her what drugs she could take for the depression that had developed following the loss of the child. In a thematic picture completed half an hour later concerning his most important memories from childhood, he drew four pregnant women ( Figure 3 ) and entitled the picture “Maternity”; he later stated:

A therapeutic drawing made by PA (the task was to draw something from childhood), which he entitled “Maternity.” Note that he has drawn two pairs of pregnant women, clearly making them into doubles. This is not because of double vision.
“They could have been my patients. But I can’t really recall whether I had such patients. When I think about it I see a black hole, such a void”.
He was also able to recognize and describe the ultra sonogram of a baby shown to him by his daughter, commenting:
“…. it’s a baby in ultrasound 3D … it could be in the 29 th or 30 th week of pregnancy. Do you think it’s that old? It looks very healthy!
Yet he protested with anger when his daughter said that this was his grandson, crying:
“… Oh no! Don’t try and pull the wool over my eyes! I can’t be a grandfather because I don’t have any children. My whole family died in an accident.
Moreover, he was able to give details of gynecological operations, but was extremely surprised to find that he could do so. He did not remember studying medicine, but was able to recognize the university building. This reflects a discrepancy between well-preserved medical knowledge and the lack of autobiographical memory. He had no recollection of having obtained a medical diploma even when it was shown to him, claiming that what he was seeing must have been counterfeit.
At the same time he proved to have preserved semantic memory, while autobiographical memory appeared to be lost. Thus he stated that people had children:
“to develop a so-called procreative family, to maintain the human species, to pass on their genes.
Yet he denied having children of his own, even when he was presented with their pictures:
“… These are children, but not mine. I’m 18 years old. I’m too young to have children, right? It’s not my blood and genes these children are carrying.
Chronic status and ongoing rehabilitation
PA has been under neuropsychiatric and neuropsychological observation for 10 years, from January 1999 to June 2009. Treatment was based on a comprehensive model, including neuropsychological rehabilitation. In neuropsychological testing we found the following symptoms:
- traces of aphasia with minor naming difficulties;
- minor problems with the production of syntactically correct sentences;
- working memory problems and severe learning problems (PA does not remember anything longer than a few minutes at the most), especially with delayed memory tasks;
- severe problems with autobiographical memory (the patient remembers very little about his life before the accident);
- persistent disturbances of actuators, e.g. the patient soaps himself in the shower and then leaves the bathroom, entering the living room naked in the presence of his children;
- features of frontal lobe syndrome;
- persistent identity impairment (PA does not recognize himself in the mirror or in photographs, though he knows his name).
- a steady decline in his IQ, as measured by the Polish version of the Wechsler Adult Intelligence Scale – Revised;
- features of “temporal syndrome” (altered consciousness);
- a high level of anxiety;
- inability to perform activities of daily living;
- bizarre behavior, with an apparent inability to conform to social norms (especially laughing or shouting at inappropriate moments, e.g. during church services).
In this context it is perhaps significant that the metabolic changes in PA’s brain, measured by HMRS, are at present close to the spectrum seen in fronto-temporal dementia (FTD) [ 9 , 10 ]. Figure 4 shows a statistically significant drop in NAA concentration, and also in the relative NAA/Cr concentration.

The HMRS Spectrum – Patient PA (below-normal NAA peak, above normal mI peak)
At the same time there is a statistically significant growth in the concentration of mI, as well as the relative mI/Cr concentration. Another important indicator is the relative relation of the NAA/mI concentrations; this value shows a statistically significant drop when compared to age- and gender-matched norms. It should be added here that the localization of pathological changes in the case of the spectrum obtained from patient PA is similar to that of patients with FTD. In PA’s case, however, these changes are localized chiefly in the right frontal lobe, and not bilaterally, as in the typical FTD patient.
The collapse of family ties
Memory disturbances cannot totally explain forgotten family ties [ 11 – 13 ]. Prior to the accident, PA’s family had relatively normal family ties, though the bond between the patient and his wife was strained by the fact that he had a lover. Now the patient does not remember that he has loved anyone, and his family feels baffled by his strange behavior. His wife, his children, and his parents say he is quite a different man than he used to be. They say it is difficult to have warm feelings towards a man who is so quarrelsome, rude, and irresponsible. They feel his presence as a stranger among them. Moreover, the bonds that united and bound the remaining members of the family to each other have also collapsed, or have been seriously shaken.
It is also plausible that the dissociation of PA’s identity may be caused, at least to some extent, by his premorbid personality traits. Interviews with his family have revealed that his mother was a dominant person who used to made all her sons to act in accordance with her will despite their own wishes. We learn that PA dreamed of being a car mechanic but he became a doctor as his mother had wished. It is of interest to note that being a car mechanic is one of his adopted personalities now. It was also noted that the patient’s attitude towards his mother underwent considerable changes. He is disobedient and shouts at her, though she shows no emotional reaction to this kind of behavior. It may be concluded, then, that she was always a self-disciplined cold mother, and this – as we know – may lead to a number of emotional disorders in the child. In fact, childhood trauma is believed to be one of the causes of Dissociative Identity Disorder [ 14 ].
Another significant trait of PA’s personality was his tendency to lie. First of all he had a number of love-affairs, which meant he had to make various stories to hide this from his wife and family. Moreover, his wife reports that he tended to tell small lies to his friends just “to make life more interesting,” to use his own words. Naturally, this does not explain the nature of PA’s disorders, but they cannot be explained solely by memory disturbances as well, though memory, especially autobiographical memory, certainly plays an important role in integrating one’s concept of self.
At the same time, both linguistic functions and acquired knowledge (including medical skills) have remained intact to a considerable degree. It is worth noting here that these are functions that do not concern PA personally. In other words, he has lost mainly the emotionally loaded information, which may explain – at least to some extent – his emotional reactions to suggestions concerning his true personal relations.
Short-term memory
PA’s memory disorders include not only autobiographical memory, but also short-term memory. Some deficits were apparent on standardized memory tests, based on recall after 20–30 minutes of filled delay, but we observed clinically that he seemed unable to recall anything at all 2 hours after the event occurred. In order to measure this, we designed a simple experiment, which consisted in choosing a particular event that occurred during the ordinary hospital day (e.g. a visitor, a meal, a physiotherapy session or the like), and then asking PA what he remembered immediately after the event, and then again at four time points: 30 minutes, 60 minutes, 90 minutes, and 120 minutes. This experiment was repeated 6 times during PA’s hospitalization at the Cracow Rehabilitation Centre in summer of 2003, and again in the summer of 2004. The amount of information recalled immediately after the event was treated as 100% for baseline, and the amount of recall at subsequent time points was calculated in terms of information units.
As shown in Figure 5 , the intensity of the effect achieved when PA records a given event in memory diminishes rapidly over time, in comparison to the effect that accompanied the event itself. The magnitude of the negative effect exceeds the dimensions of the positive effect. Considering the automatic scaling on the graph, the differences at a time of storage of 1.5 hours appear to be slight, but these differences – like the others – are statistically significant.

Recollection of a given event in the neuropsychological examination of short-term memory in patient PA.
Two examples follow:
On one occasion, according to hospital schedule, PA was sent to kinesitherapy for 2 hours. When he returned to the ward, we asked PA what had happened, and he gave a reasonably full account of the exercises he had been through, which coincided in all essentials with the physiotherapist’s report. When asked for the same information 30 minutes later, some details were missing, but the essentials were in place. At one hour, however, he remember only the last half-hour of exercises, and insisted that he had been there only half an hour. His account at 90 minutes was extremely sketchy, and there were some confabulations. At two hours, he denied that he had ever been in physiotherapy. He did remember that he had already been asked several times for that information, but became agitated that we were pestering him about something that never happened. He began to express indignation that no one had remembered to take him to kinesitherapy, and all attempts to convince him that he had actually been there met with an increasingly aggressive reaction.
On another occasion, nearly a year later, he was visited one day by his wife, who spent an hour with him on a day when she usually was unable to come see him. Immediately after she left, he gave a reasonably full account of the event, which his wife later verified as substantially accurate. Within half an hour, he remembered only that his wife had “probably” been there, though he expressed uncertainty as to whether this had not happened the day before. At 90 minutes he remembered that someone had been there, but not who. At two hours he was insisting that he had been alone all day, with no visits.
Thus memory traces of emotionally laden material, both positive and negative, tend to diminish and disappear completely after a period of two hours. This would not be especially surprising, if the material forgotten consisted of a list of random numbers or words, or even a story read aloud by an examiner, as in most standard memory tests. In fact, however, PA forgets everything that happens to him, no matter how much it matters to him. Moreover, he does not remember that he has forgotten something, a state which occurs only in medium-to-late stages of dementia. This means that both long-term and short-term memory are severely disturbed.
What makes the case described above nearly unique is the progression of symptoms, which seem to cover an entire spectrum of memory disturbances. At any given moment, the mosaic of PA’s memory deficits and retained capacities could be accounted for in the familiar terms of classical neuropsychology, as a set of disturbances resulting from damage to a particular module or memory system (short-term, long-term, episodic, semantic, and so forth). If PA were to be included in a large group study of severe TBI patients, his scores at any given moment would enable him to be assigned to a sub-group without any particular difficulty. The problem is that if the same tests were administered only a few months (or even weeks) later, he would just as easily be assigned to another, quite different group, at a moment when he seems to have regained some aspects of memory, while losing his grip on something he had previously had under control.
Yet it does not suffice merely to superimpose a “typical” neurodegenerative process on a “typical” focal lesion to produce a picture resembling PA’s symptoms. Though it is not impossible that FTD is developing in PA’s brain independently of the TBI, this would be a most remarkable coincidence, and in any event the course of both diseases would certainly be profoundly affected by the presence of the other. In order to understand PA’s case properly, it is essential to look at what has happened to him, not as “status post,” i.e. a particular event (cause, i.e. the accident) with its various sequelae (effect, i.e. the symptoms), and not even as a disease, where the onset of illness (the cause) is assumed to set in train a cascade of events (the symptoms), but rather as a process (the patient’s life as a whole), whose course has been radically altered by an event. This is illustrated by Figure 6 .

A schematization of changes in trajectory caused by a sudden event
For the present purposes, let segment A-B represent the course of PA’s life, where A represents the starting point and B the goal to which PA aspires. The appearance of a strong external force (C, here representing the accident) pushes the vector in a different direction, to his current status, represented as D. What is most important here is that the vector C-D is not simply the result of the direction and strength of C, but rather the effect of C on the original vector A-B.
The fluctuation of syndromes observed in PA can be best explained by microgenetic theory. The syndrome described here results from the unfolding of the lower layers of the process of becoming, from core (self) to perception (world), which frames the mind/brain state. Consciousness is the relation of early to late or depth to surface in this process [ 15 , 16 ]. Visual and verbal imagery, including conceptual or intentional feeling, arise at intermediate phases, so long as an external world is realized. The arrow in Figure 7 represents sensation acting on the phase of imagery to externalize and adapt the state to the physical world. The phase-transition is non-temporal until it terminates. The mind/brain state and immediate present develop in a fraction of a second, replaced by overlapping states.

The transition, or process of becoming, from core (self) to perception (world) frames a mind/brain state. Consciousness is the relation of early to late or depth to surface in this process. Visual and verbal imagery, including conceptual or intentional feeling, arise at intermediate phases, so long as an external world is realized. The arrow represents sensation acting on the phase of imagery to externalize and adapt the state to the physical world. The phase-transition is non-temporal until it terminates. The mind/brain state and immediate present develop in a fraction of a second, replaced by overlapping states [ 15 ].
As mentioned in the introduction by Brown and Pachalska [ 17 ], a traditional approach to symptoms observed after brain lesions does not provide an explanation for the change observed in clinical syndromes in the course of recovery or deterioration. It provides support for the explanation of the symptom according to microgenetic theory.
This case raises the question of the origin of:
- stability, whenever the world and mind are in constant change;
- the nature of identity: is it continuous?
According to Marcia [ 18 ] there are four phases in the process of forming an identity:
- In diffuse identity the self is vaguely defined and ill-formed. There is no way even to ask the question, “Who am I”, let alone answer it. The first person singular does not exist, either as a grammatical function or as a psychological category, only a kind of primordial chaos of undifferentiated being [ 19 , 20 ].
- In mirror recognition, the individual recognizes her/himself only in others, especially parents. The individual plagiarizes a self perceived as being their own self. There is no chance for making independent choices that would not be consistent with the choices made by the significant other, whose reflection is one’s own self.
- In deferred identity, the maturing self becomes aware of its otherness, and at the same time of its incompleteness, which it strives to fill with selected contents. There is an attempt to distance oneself from the previous points of identification, replacing them with new ones, as teenagers rebel against their parents and begin to identify themselves with other kinds of groups.
- In mature identity there is a delicate balance maintained between a sense of belonging and a sense of one’s own uniqueness, between continuity and change.
These phases are typically presented as phases in human development over one’s lifespan, but in the case of our patients it is possible to see each of them emerging after coma through all four phases. In akinetic mutism and the phases that follow, the patient shows no willful behavior in spite of possessing a working nervous system, central and peripheral, primarily because there is no consciousness that action as such exists or is possible.
Our case shows that a self is not a persistent object but a recurrent state. Hume (1740/1967: Book I, Part IV, Section VI) [ 21 ] questioned the existence of identity, which he believed to be an illusion, and so he could not account for the sense of identity over time [ 22 ].
James [ 23 ] as well as Whitehead [ 24 ] were of the opinion that identity was due to an overlap of mental states. Self has to be created in each moment, and this creative process leads to a more dynamic way of thinking about the mind and brain [ 25 ].
Microgenetic theory provides an account of the process of creating the self [ 26 – 34 ]. It emphasizes the span of neural time (in microseconds in the case of a healthy adult) that on the one hand enables continuity of the self, and on the other buffers it from falling apart [ 35 – 37 ]. The way the self is structured is shown in Figure 8 . There is a minimal self, the irreducible core, whose existence is necessary in order for the organism to be an organism, and not merely a collection or colony of cells [ 38 ]. We feel this minimal self in our most fundamental biological/mental state, the awareness of being “right here right now” and having some kind of experience. A longitudinal self arises when memory allows the discrete moments of “minimal self” time to be bundled in larger units, making it possible not only to feel one’s existence in the present moment, but also to conceive of that existence in the past and imagine it continuing into the future.

The interconnections among the self and the minimal and longitudinal self.
The case of PA demonstrates that the minimal self can persist, stranded in the moment, without the privilege of mental time travel that episodic memory affords. An integrated longitudinal self, however, requires alignment of one’s current mental state and enduring semantic knowledge of personal traits, goals, beliefs, and values. It requires seamless access to the episodic memory of past and present selves that ground semantic self knowledge and infuse it with emotional meaning.
In other words, a person whose identity is stable needs to be:
- aware of past, present, and future;
- able to perceive her surroundings;
- able to access her memories;
- able to operate with reasons, i.e. conceive several options, select one of them as better than the others, and realize it;
- capable of making decisions;
- responsible for at least some of her own behavior.
Unfortunately, all those conditions are not present in the patient described here, which resulted in the gradual loss of the sense of personal identity. His case, however, allows one to draw a hypothesis on the way the self has to be buffered in order to protect it from disintegration.
The process of insulating the self is secured due to the expansion from the core self into the subjective “I”, then the formation of an object world distinct from the “I”, which thus preserves its integrity and identity through time – or at least for some segment of time.
There is a paradox in all this, which is that the subjective self, having created an objective world and separated itself from it, at the same time becomes conscious of itself as an object. The “I” can conceive of, evaluate, look at, analyze, interpret the “me”, the self seen by the subject as an object among other objects. This distinction between the “I” and the “me” makes possible not only introspection, but also social thinking, the ability to conceive of oneself as a human object among other human objects, without losing the “I” – or at least without the necessity to lose it, though the risk is certainly there.
The distinction between “I” and “me” is more on the order of actual and potential than knower and known [ 39 ]. The knowing self – the minimal “I” at the threshold of consciousness - knows that which develops out of it. The subliminal “me” that remains beneath as the potential for the ‘I’ is implicit, unconscious and inaccessible. It represents, or is part of, the tacit knowledge of the individual, what the person knows or has the capacity (competence) to know, and it gives rise to the conscious self, to thought and action. The known self is not actually known, it is felt, intuited, sought after. It participates covertly in thought, but is not ultimately revealed.
Variability of syndromes
The changes that have occurred in the clinical picture of PA’s mental status are perplexing. He has passed through a mosaic of disturbances, which resulted in a continuous deterioration of his identity:
- Misidentification (person and place);
- Capgras syndrome for person(s);
- Capgras for environment;
- Capgras for the arm (asomatognosia);
- Frégoli syndrome for person(s);
- Frégoli for environment;
- Delusional reduplication (without misidentification) of self or other persons;
- Loss of identity (Cotard’s syndrome, the delusion of being dead).
As illustrated in Figure 9 , time has played an important role in the process of PA’s continuous deterioration. In fact, he has completely lost orientation in his surroundings, and his attempts to discover his true identity have ceased.

Development of syndromes in patient PA over time.
In a recent publication, the first author of the present study has described these changes in self-identity, which include many, if not most, of the syndromes of impaired recognition of self and others described in the literature [ 40 , 41 ]. The problem of personality and the self-concept can be approached, from the standpoint of pathology, in terms of patterns of transition from one symptom-complex to another in the same individual, and not as isolated defects in particular individuals within a population. Disorders of the self cannot be localized to separate brain areas, but constitute a spectrum in the process through which personality is preserved and sustained. The case described here provides convincing evidence that the stability and identity of the self depends, not on the association of discrete components, but on a recurrent process that maintains the self-concept over time, in aging, through sleep, and in the course of changing life events.
Time, perception, and self
As noted elsewhere by the first author of the present study [ 41 , 42 ] the basic components of identity, those that can be weakened or destroyed by brain damage, are the following:
- coherence , that is, the idea that being oneself makes some kind of sense, that the parts which make up the whole fit together, at least in a general way;
- unity , that is, the requirement that fundamentally there is one self in one body, despite the complexity of its structure;
- continuity , that is, the requirement that the coherence and unity of identity last as long as life itself lasts, from birth to death, despite the natural tendency to break life down into periods (epochs, eras).
It turns out that one of PA’s problems is the loss of the feeling of time, due mainly to the disturbances of autobiographic memory [ 43 ]. MacQueen [ 39 ] has suggested that when speaking of that type of memory we concentrate on “auto-”, and forget about the original meaning of “biography.” The ancient Greek word bios meant “life,” and grapho meant “to write.” Our autobiography is therefore essentially a story, composed of the events of our own life, and is therefore narrative in its very nature. It is not, however, an orderly and exhaustive story that includes literally all the events of our life, but rather a kind of sketch made up of only those events which are of importance to us. As a rule these are emotionally loaded events, as well as those which proved to be of vital consequence for our future. In this respect autobiographic memory is more like a play or a film, in which significant events (episodes, scenes, moments) are combined in a way to form a comprehensive and continuous story. The story gains its coherence due to the logical sequence of events, where the viewer is left to assume or infer a logical sequence from one event to the next. In the case of the autobiography (understood here as a mental construct, not a literary genre), such a sequence of events is ensured by our memory. Thus identity problems in TBI patients should hardly be surprising. Even if the sequence of symptoms presented by PA seems unique, the complaint that one is “a different person” after the injury is not [ 41 , 42 ].
This means that both long-term and short-term memory are severely disturbed. In consequence, he lives only in the present, since both past and future have ceased to exist for him. As pointed out by Brown [ 43 ], the past is an essential component of the feeling of the present, which develops out of the immediate state revived in the present moment, while the future does not exist other than as an idea, or a feeling of the surge forward to the present [ 30 ]. No wonder PA has lost his sense of self, as he has no elements to refer to.
To make matters worse, he also encounters difficulties in evaluating the surrounding world, which is blurred and difficult to comprehend. It is worthy to remind here that we create our picture of current reality on the basis of our previous knowledge and experience [ 40 ], and PA has lost an access to them. According to microgenetic theory the primary activity of mind is to ‘chunk’ experience into private and public objects or events [ 27 – 29 ]. In other words, we are able to perceive (or recognize) only those objects and events that correspond to models created in our mind by experience. The models sculpt a complex reality into meaningful and comprehensible wholes [ 44 ]. As Brown [ 15 ] puts it: “The inner connectedness of the world is not its ostensible relatedness in the world, but its formative trajectory in the mind/brain.” (p. 251).
PA is not able to “chunk” his experience, to create meaningful units out of the continuous flow of stimuli he is confronted with. Hence, the world around him is chaotic and incomprehensible, and he is an observer, whose only function is to react to situations he does not understand. In consequence he has no means to form his self-awareness. Only his core self, acting at the limbic, unconscious level is intact. This is reflected in his emotional reactions to any attempt to make him realize who he really was, as well as reactions to music he formerly liked. In a way, there is a regression to a former state of consciousness, which may also explain why he has stopped smoking and drinking: after all, these are not the activities of the boy he believes himself to be.
Acting on the limbic level explains also his inability to control his emotions and his inappropriate social behaviors. But most disastrous is the fact that he has lost his identity, since he has no elements to rely upon. Hence, he tends to cling to the self of others, borrowing their identities at least for the period he is able to remember.
Additional problems are created by frontal lobe dysfunction, which makes it difficult – if not impossible – for PA to assemble the disparate pieces of his foggy world.
Acknowledgment
We want to express our gratitude to those friends who have patiently heard us out on many of these topics and offered their own insightful opinions. They include Prof. Danuta Kadzielawa, Prof. Marek Moskala and Prof. David Bradford. We especially want to express our gratitude to our dear friend Prof. Jason W. Brown for his advice, support and inspiration over the years.
Source of support: Departmental sources
Applying the Consensus Criteria for Traumatic Encephalopathy Syndrome Retrospectively to Case Studies of Boxers from the 20th Century
Affiliations.
- 1 Department of Physical Medicine and Rehabilitation, Spaulding Rehabilitation Hospital, Charlestown, Massachusetts, USA.
- 2 Department of Physical Medicine and Rehabilitation, Harvard Medical School, Boston, Massachusetts, USA.
- 3 Department of Physical Medicine and Rehabilitation, Schoen Adams Research Institute at Spaulding Rehabilitation, Charlestown, Massachusetts, USA.
- 4 Sports Concussion Program, Mass General for Children, Boston, Massachusetts, USA.
- 5 Home Base, A Red Sox Foundation and Massachusetts General Hospital Program, Charlestown, Massachusetts, USA.
- 6 Sydney School of Health Sciences, Faculty of Medicine and Health, The University of Sydney, Camperdown, New South Wales, Australia.
- 7 Department of Pathology, Northwestern University Feinberg School of Medicine, Chicago, Illinois, USA.
- PMID: 38595792
- PMCID: PMC11002329
- DOI: 10.1089/neur.2023.0134
There are no validated diagnostic criteria for traumatic encephalopathy syndrome (TES). During the early and middle 20th century, TES was described as a clinical condition that was experienced by some high-exposure boxers-and it was believed to reflect chronic traumatic brain injury. Consensus criteria for the diagnosis of TES were published in 2021. We applied the consensus criteria for TES retrospectively to cases of chronic brain damage in boxers described in articles published in the 20th century that were obtained from narrative and systematic reviews. The sample included 157 boxers identified in 21 articles published between 1929 and 1999. Two authors reviewed each case description and coded the criteria for TES. For the core clinical features, cognitive impairment was noted in 63.1%, and in 28.7% of cases the person's cognitive functioning appeared to be broadly normal. Neurobehavioral dysregulation was present in 25.5%. One third (34.4%) were identified as progressive, 30.6% were not progressive, and the course could not be clearly determined in 35.0%. In total, 29.9% met the TES consensus criteria, 28.0% did not, and 42.0% had insufficient information to make a diagnostic determination. TES, in the 20th century, was described as a neurological condition, not a psychiatric disorder-and this supports the decision of the 2021 consensus group to remove primary and secondary psychiatric diagnoses from being a core diagnostic feature. Future research is needed to determine whether, or the extent to which, cognitive impairment or neurobehavioral dysregulation described as characterizing TES are associated with chronic traumatic encephalopathy neuropathological change.
Keywords: chronic traumatic encephalopathy; concussion; dementia; neurological disorders; sports; traumatic brain injury.
© Grant L. Iverson et al., 2024; Published by Mary Ann Liebert, Inc.
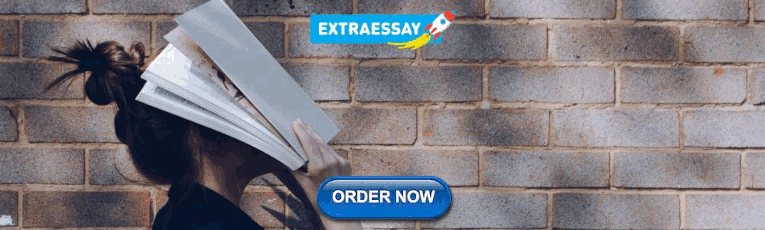
IMAGES
VIDEO
COMMENTS
In the United State alone, there are approximately 1.5 million traumatic brain injuries (TBI) per year, and TBI is the leading cause of death among individuals under the age of 45 [ 1, 2 ]. Annually, these injuries result in approximately 50 000 deaths and about 80 000-90 000 cases of debilitating head injuries [ 2 ].
Background. Traumatic brain injury (TBI) is a key cause of death and disability in young adults and is becoming more prevalent. 1 While advances in prehospital care have reduced all-cause mortality following major trauma, a reduction in mortality following TBI has not occurred since 1994. 1 Intracranial pressure (ICP) and cerebral perfusion ...
The aim of this study was to identify which drugs are currently being evaluated in clinical trials for TBI. A search of ClinicalTrials.gov was performed on 3 December 2021 and all clinical trials that mentioned "TBI" OR "traumatic brain injury" AND "drug" were searched, revealing 362 registered trials. Of the trials, 46 were ...
In this case report, we demonstrate the unanticipated recovery of a 28-year-old male patient who presented with a severe traumatic brain injury after being in a motorcycle accident without wearing a helmet. He presented with several exam and imaging findings that are statistically associated with increased mortality and morbidity.
traumatic brain injuries (TBI) per year, and TBI is the leading. cause of death among individuals under the age of 45 [1,2]. Annually, these injuries result in approximately 50 000 deaths. and ...
Conclusions and Relevance: This case study investigates the structural effects of traumatic brain injury for the first time using pre-injury and post-injury 7 Tesla MRI longitudinal data. We report findings of initial volumetric changes, decreased structural connectivity and reduced microstructural order that appear to return to baseline 8 ...
Abstract. TBI rehabilitation case is a case example of traumatic brain injury (TBI) using neuropsychological (NP) and neurological evaluations and follow-ups to assist the patient in helping her reach her long-term rehabilitation goals. The largest obstacles to achieving success included the patient's defensiveness and psychological reactions ...
Providing care for a critically ill patient with multi-trauma and traumatic brain injury (TBI) in an intensive care unit (ICU) requires that clinicians understand the mechanisms of injury and potential consequences of clinical decision making. ... In Part I of this case study, Peter, 32, was admitted to hospital with multiple injuries including ...
Background: The burden of traumatic brain injury (TBI) poses a large public health and societal problem, but the characteristics of patients and their care pathways in Europe are poorly understood. We aimed to characterise patient case-mix, care pathways, and outcomes of TBI. Methods: CENTER-TBI is a Europe-based, observational cohort study, consisting of a core study and a registry.
This case study describes the management of a 54-year-old male who presented to the Hospital of the University of Pennsylvania (HUP) with a traumatic brain injury (TBI) after being assaulted. He underwent an emergent bifrontal decompressive hemicraniectomy for multiple, severe frontal contusions. His postoperative course included monitoring of ...
Tel.: +61 2 95 144 804; fax: +61 2 9514 4835. E-mail address: [email protected] (S. Ladanyi). Traumatic brain injury (TBI) is a leading cause of death and injury worldwide. This cohort of patients present a multitude of complex clinical challenges to critical care nurses and demand com-.
Traumatic brain injury (TBI) has the highest incidence of all common neurological disorders, and poses a substantial public health burden. TBI is increasingly documented not only as an acute condition but also as a chronic disease with long-term consequences, including an increased risk of late-onset neurodegeneration. The first Lancet Neurology Commission on TBI, published in 2017, called for ...
1. Introduction. Traumatic brain injury (TBI) is a disruption in normal brain function caused by external mechanical force, such as rapid acceleration or deceleration, a bump or jolt to the head, or penetration by a projectile. As an acquired brain injury (i.e., postnatal brain damage), TBI is differentiated from nontraumatic brain injuries not ...
TRAUMATIC BRAIN INJURY CASE STUDIES This project was supported by funding from the National Institutes of Health Blueprint for Neuroscience Research under grant #R25DA033023 and additional funding from the Dana Foundation. Its content is solely the responsibility of the authors and does not necessarily represent the official views of NIH or the ...
Traumatic brain injury (TBI) can be defined as an alteration in brain functioning, or evidence of pathology within the brain, caused by an external force such as a jolt or blow to the head. l . An injury to the head can result in a TBI with the primary causes of TBIs including falls, motor vehicle crashes, struck by or against objects, and ...
In this case-control study of military-related mTBI, our results showed that DTI and NODDI can detect microstructural white matter alterations in the chronic phase of mTBI, implicating inflammatory and neurodegenerative processes. ... Diffusion tensor imaging studies of mild traumatic brain injury: a meta-analysis. J Neurol Neurosurg ...
Read chapter Case Study 1 of Improving Functional Outcomes in Physical Rehabilitation, 2e online now, exclusively on F.A. Davis PT Collection. F.A. Davis PT Collection is a subscription-based resource from McGraw Hill that features trusted content from the best minds in PT. ... Cowden T.T. Cowden, Temple T. Patient With Traumatic Brain Injury ...
Introduction. Traumatic Brain Injury (TBI) plays a significant role in morbidity and mortality within the United States, encompassing a range of injuries affecting brain function due to an external force to the skull ().Approximately 2.8 million head injuries occur each year in the United States alone, with skull fracture occurring in 28-37% of those diagnosed with TBI (2, 3).
The present study evaluated the effectiveness and perceived efficacy of the newly developed customized tele-cognitive rehabilitation programme for three subjects with traumatic brain injury through using single-case and qualitative research design, and showed improving trends and levels of specific cognitive performance during the treatment phase.
INTRODUCTION. Traumatic brain injury (TBI) is defined as changes in brain function caused by an external force. It is an important cause of death or severe physical and mental dysfunction, leading to social and economic losses [].Approximately 69 million new TBI cases have been reported worldwide each year [].About 480,000 new cases of TBI occur annually in Korea, and the total medical cost of ...
Introduction. Traumatic brain injury (TBI) has a high prevalence in adults and children and is a major cause of mortality and morbidity. In the pediatric population, mild TBI (mTBI) is the most frequent form of brain injury, with ~80% of the cases and it is reported with an incidence between 47-280 per 100,000 children (Dewan et al. 2016; Taylor et al. 2017).
Traumatic brain injury is the leading cause of death and disability in children and young adults in the United States. It is also a major concern among people over 75, with high rates of death and hospitalization. 1 Common mechanisms of injury over all age groups include motor vehicle crashes, falls, injuries from firearms, and assaults. It has been a prevailing thought that there is little ...
But in this case, the traumatic brain injury itself causes the blood to leak into the brain. The new study showed, for the first time, that fibrin is responsible for turning good immune cells bad ...
BACKGROUND. Over 1.7 million people present to US emergency departments (EDs) after sustaining traumatic brain injury (TBI) each year.[] Of those, approximately 52 000 will die, 275 000 are hospitalized, and 1.4 million are evaluated and discharged from an EDs.[] Distribution of age is trimodal with predominance in the age groups of: 0-4 years; 15-19 years; and >65 years.
Case Report. We describe progressive deterioration in personal identity in a former physician who had sustained a serious head injury (1998), resulting in focal injuries to the right frontal and temporal areas. He regained consciousness after 63 days in coma and 98 days of post-traumatic amnesia, but has since displayed a persistent loss of ...
MSN
There are no validated diagnostic criteria for traumatic encephalopathy syndrome (TES). During the early and middle 20th century, TES was described as a clinical condition that was experienced by some high-exposure boxers-and it was believed to reflect chronic traumatic brain injury. Consensus criteria for the diagnosis of TES were published in ...