An official website of the United States government
Official websites use .gov A .gov website belongs to an official government organization in the United States.
Secure .gov websites use HTTPS A lock ( Lock Locked padlock icon ) or https:// means you've safely connected to the .gov website. Share sensitive information only on official, secure websites.
- Publications
- Account settings
- Advanced Search
- Journal List

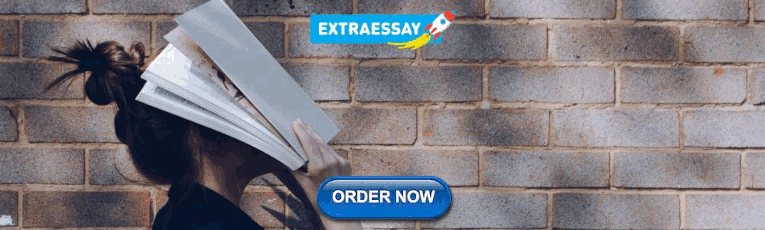
Bioinformatics approaches and applications in plant biotechnology
Yung cheng tan, asqwin uthaya kumar, ying pei wong, anna pick kiong ling.
- Author information
- Article notes
- Copyright and License information
Corresponding author.
Received 2021 Nov 30; Accepted 2022 Jul 5; Collection date 2022 Dec.
Open Access This article is licensed under a Creative Commons Attribution 4.0 International License, which permits use, sharing, adaptation, distribution and reproduction in any medium or format, as long as you give appropriate credit to the original author(s) and the source, provide a link to the Creative Commons licence, and indicate if changes were made. The images or other third party material in this article are included in the article's Creative Commons licence, unless indicated otherwise in a credit line to the material. If material is not included in the article's Creative Commons licence and your intended use is not permitted by statutory regulation or exceeds the permitted use, you will need to obtain permission directly from the copyright holder. To view a copy of this licence, visit http://creativecommons.org/licenses/by/4.0/ .
In recent years, major advance in molecular biology and genomic technologies have led to an exponential growth in biological information. As the deluge of genomic information, there is a parallel growth in the demands of tools in the storage and management of data, and the development of software for analysis, visualization, modelling, and prediction of large data set.
Particularly in plant biotechnology, the amount of information has multiplied exponentially with a large number of databases available from many individual plant species. Efficient bioinformatics tools and methodologies are also developed to allow rapid genome sequence and the study of plant genome in the ‘omics’ approach. This review focuses on the various bioinformatic applications in plant biotechnology, and their advantages in improving the outcome in agriculture. The challenges or limitations faced in plant biotechnology in the aspect of bioinformatics approach that explained the low progression in plant genomics than in animal genomics are also reviewed and assessed.
There is a critical need for effective bioinformatic tools, which are able to provide longer reads with unbiased coverage in order to overcome the complexity of the plant’s genome. The advancement in bioinformatics is not only beneficial to the field of plant biotechnology and agriculture sectors, but will also contribute enormously to the future of humanity.
Keywords: Bioinformatics, Biotic and abiotic, GWAS, NGS, Plant breeding, Plant sequencing, Plant pathogen, PRGdb sequence analysis
Over the past decades, the term ‘bioinformatics’ has become a buzzword in all areas of research in biological science. With the continuous development and advancement in molecular biology, the explosive growth of biological information required a more organized, computerized system to collect, store, manage, and analyse the vast amount of biological data generated in the experiments from all fields [ 1 ]. Bioinformatics, as a new emerging interdisciplinary field for the past few decades, has many tools and techniques that are essential for efficient sorting and organizing of biological data into databases [ 1 , 2 ]. Bioinformatics can be referred as a computer-based scientific field which applies mathematics, biology, and computer science to form into a single discipline for the analyses and interpretation of genomics and proteomics data [ 2 , 3 ]. In short, the main components of bioinformatics are (a) the collection and analysis of database and (b) the development of software tools and algorithm as a tool for interpretation of biological data [ 2 ]. Bioinformatics played a crucial role in many areas of biology as its applications provide various types of data, including nucleotide and amino acid sequences, protein domains and structure as well as expression patterns from various organisms [ 3 ]. Similarly, the field of plant biotechnology has also taken advantages of bioinformatics, which provides full genomic information of various plant species to allow for efficient exploration into plants as biological resource to humans [ 1 , 3 , 4 ]. The intention of this article is to describe some of the key concepts, tools, and its applications in bioinformatics that are relevant to plant biotechnologies. The current challenges and limitations for improvement and continuous development of bioinformatics in plant science are also described.
Applications of bioinformatics in plant biotechnology
The introduction of bioinformatics and computational biology into the area of plant biology is drastically accelerating scientific invention in life science. With the aid of sequencing technology, scientists in plant biology have revealed the genetic architecture of various plant and microorganism species, such as proteome, transcriptome, metabolome, and even their metabolic pathway [ 1 ]. Sequence analysis is the most fundamental approach to obtain the whole genome sequence such as DNA, RNA, and protein sequence from an organism’s genome in modern science. The sequencing of whole genome permits the determination of organization of different species and provides a starting point to understand their functionality. A complete sequence data consists of coding and non-coding regions, which can act as a necessary precursor for any functional gene that determines the unique traits possessed by organisms. The resulting sequence includes all regions such as exons, introns, regulator, and promoter, which often leads to a vastly large amount of genome information [ 5 ]. With the emergence of next-generation sequencing (NGS) and some other omics technologies used to examine plants genomics, more and more sequenced plants genome will be revealed [ 1 , 6 – 8 ]. To deal with these vast amounts of data, the development and implementation of bioinformatics allow scientists to capture, store, and organize them in a systematic database [ 1 , 5 ].
Bioinformatics databases and tools for plant biotechnology
In the field of bioinformatics, there are a variety of options of databases and tools that are available to perform analysis related to plant biotechnology. Next-generation sequencing (NGS) and bioinformatics analysis on the plant genomes over the years have generated a large amount of data. All these data are submitted to various and multiple databases that are publicly available online. Each database is unique and has its focus. For instance, CottonGen, database is solely dedicated to obtaining genomics and breeding information of any cotton species of interest [ 9 ]. The establishment of such database eases the researchers who are working on cotton genomic studies by focussing on using just one database instead of searching through other available databases. However, some databases are established and designed to cater not only to one specific species or genus, but focus on all the plant species, such as the National Center for Biotechnology Information (NCBI) ( https://www.ncbi.nlm.nih.gov/ ) database, which as of 2021 possesses almost 21,000 plant genomes that are available for access [ 10 ]. Such a database is useful for studies that do not focus on one specific genus or species. This eases the researchers in accessing to all kinds of genomic data in one database. This section will briefly discuss some of the available plant genome databases, which are publicly accessible and not designated for one genus or species alone.
First would be the globally known and recognized database by all the researchers and biologists, which is the NCBI database. NCBI has been dedicated for gathering and analysing information about molecular biology, biochemistry, and genetics. In the NCBI database, one can download the genome information of the plant species of interest from either gene expression omnibus (GEO) ( https://www.ncbi.nlm.nih.gov/geo/ ) or sequence read archive (SRA) ( https://www.ncbi.nlm.nih.gov/sra ) by simply stating the scientific name of the plant in the search bar and the entire genomic information of the plant can then be obtained. The GEO and SRA comprise processed or raw gene expression data or RNA sequencing of plants that are reposited in the repository. For instance, to obtain the genomics of Rosa chinensis (Rose plant), by inputting the name in the search bar, it will direct to the search result page where the researcher can select the most recent or suitable datasets with specific accession number. Depending on the profiling platform used in each dataset, researchers could retrieve either gene symbols, Ensemble ID, open reading frame, chromosomal location, regulatory elements, etc. The information allows researcher to further analyse the subject of study using bioinformatics tools such as gene ontology ( http://geneontology.org/ ), Database for Annotation, Visualization and integration Discovery (DAVID) ( https://david.ncifcrf.gov/ ), Basic Local Alignment Search Tool (BLAST) ( https://blast.ncbi.nlm.nih.gov/Blast.cgi ), and others that is relevant for the study.
Another database that is available for accessing plant genome database is EnsemblPlants ( https://plants.ensembl.org/index.html ). Unlike the NCBI database, which is not only dedicated to plant genomes, EnsemblPlants is specifically dedicated to accessing plant genomes. EnsemblPlant is part of the Ensembl project that started in 1999, where the project aimed to automatically annotate the genome and integrate the outcome of the annotation with other publicly available biological data and establish an open access archive or database online for the use of the research community [ 11 ]. Ensembl project later launched the taxonomic specific websites designated for each taxon under their project that also includes the plants. The database is a user-friendly integrative platform, where it is continuously updated with the new addition of plant species every time a plant genome is completely sequenced. Compared to the NCBI database mentioned earlier, EnsemblPlant not only provides genome sequence, gene models, and functional annotation of the plant species of interest, but also includes the polymorphic loci, population structure, genotype, linkage, and phenotype information [ 11 , 12 ]. Unlike, NCBI, EnsemblPlant does also provide comparative genomics data of the plant species of interest. This indicates that the platform does not only offer genome sequence data but provide additional analytical data about the plant species of interest and help the researchers who are working on plant bioinformatics to save a lot of time by reducing the tedious work in running the analysis. Yet, the researchers could re-assess the data if necessary, depending on the stringency of their work.
Aside from the abovementioned databases that are widely used for retrieving plant genome sequence, there are still other plant databases such as PlantGDB, MaizeDIG, and Phytozome that can also be considered. Table 1 lists the available database and tools that are widely applied in plant biotechnology.
List of bioinformatics databases and tools applied in plant biotechnology
Biotechnology and bioinformatics for plant breeding
Plant breeding can be defined as the changing or improvement of desired traits in plants to produce improved new crop cultivars for the benefits of humankind [ 8 ]. Jhansi and Usha [ 13 ] mentioned a few benefits brought by genetically engineered plants such as improved quality, enhanced nutritional value, and maximized yield. The revolution of life science in molecular biology and genomics has enabled the leaps forward in plant breeding by applying the knowledge and biological data obtained in genomics research on crops [ 6 , 8 , 13 ]. In modern agriculture, transgenic technology on plants refers to genetic modification, which is done on plants or crops by altering or introducing foreign genes into the plant, to make them useful and productive and enhance their characteristic [ 13 , 14 ]. As mentioned above, the evolution of next-generation sequencing (NGS) and other sequencing technologies produces a large size of biological data which require databases to store the information. The accessibility of whole genome sequences in databases allows free association across genomes with respect to gene sequence, putative function, or genetic map position. With the aid of software, it is possible to formulate predictive hypothesis and incorporate the desired phenotypes from a complex combination into plants by looking at those genetic markers which score well and gives a higher reliability in breeding [ 2 , 15 ]. Other than genome sequence information, databases which store the information of metabolites also play a crucial role in the study of interaction with proteomics and genomics to reflect the changes in phenotype and specific function of an organism [ 1 ]. Some of the most widely used metabolomics databases for plants and crops such as Metlin ( http://metlin.scripps.edu ), provides multiple metabolite searching and about 240,000 metabolites, nearly 72,000 high-resolution MS/MS spectra, and PlantCyc ( https://plantcyc.org/ ), a database which stores information about biochemical pathway and their catalytic enzyme and genes from plants [ 1 , 16 ]. Moreover, single-nucleotide polymorphism markers also benefit from the revolution of NGS and other sequencing technologies. By using NGS, RNA sequencing (RNA-seq) allows direct measure of mRNA profile in order to identify known single-nucleotide polymorphism (SNP) [ 1 ]. SNP is the unique allelic variation within a genome of same species, which can be used as biological markers to locate the genes associated with desired traits in plants [ 17 , 18 ]. Besides, transcriptome resequencing using NGS allows rapid and inexpensive SNP discovery within a large, complex gene with highly repetitive regions of a genome such as wheat, maize, sugarcane, avocado, and black currant [ 17 ]. Figure 1 illustrates briefly the process involved in plant breeding using NGS and bioinformatics.
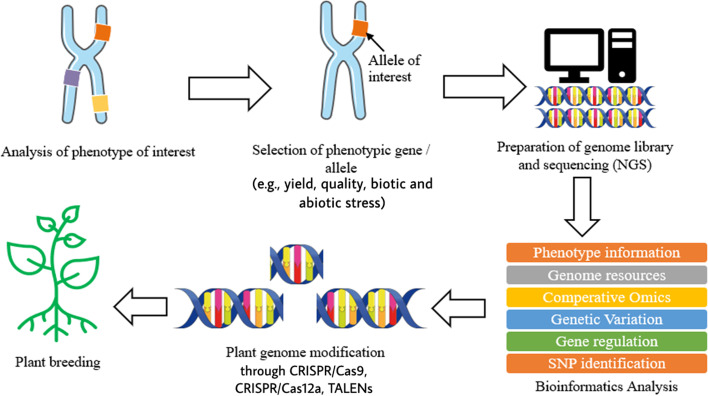
Brief process of plant breeding involving NGS and bioinformatics
Ever since the first transgenic rice production in 2000, there has been a significant revolution in crop genome sequencing projects, along with the advancement in technologies, rapidly increasing the pace in genetically modified organism (GMO) [ 2 , 13 , 19 ]. Among all the products in rice biotechnology, one of the most widely known GM rice is golden rice. Golden rice is a variety of rice engineered by introducing the biosynthetic pathway to produce β-carotene (pro-vitamin A) into staple food in order to resolve vitamin A deficiency. The World Health Organization has classified vitamin A deficiency as public health problem as it causes half a million of children to childhood blindness [ 13 ]. Vitamin A is an essential nutrient to humans as it helps with development of vision, growth, cellular differentiation, and proliferation of immune system; insufficient intake of vitamin A may lead to childhood blindness, anaemia, and reduced immune responsiveness against infection [ 20 ]. Being the first crop genome to be sequenced, rice has become the most suitable model to initiate the development and improvement of other species in genomic aspect [ 21 – 24 ]. The particular reason is due to its small genome size and diploidy, which enables rice to be an excellent model for other cereal crops with larger genomes, such as maize and wheat [ 21 , 23 ]. Song et al. [ 22 ] reported the complete genome sequence of two rice subspecies, japonica and indica , in 2005 that laid a strong foundation for molecular studies and plant breeding research [ 22 , 24 ]. With recent advancement in bioinformatics, it is now possible to run the sequence alignment between large and complex genome from other crop species with genomic data available from rice, by using different software or tools, in order to find out the shared conserved sequence through comparative genomics [ 2 , 7 ]. Vassilev et al. stated some of the most commonly used programmes such as BLAST and FASTA format allowed rapid sequence searching in databases and give the best possible alignment to each sequence [ 25 ]. The programming algorithm calculates the alignment score to measure the proportion of homology matching residue between sequence from related species [ 2 ].
Wheat, as the most widely grown consumed crops, together with rice and maize contributes more than 60% of the calories and protein for our daily life [ 26 , 27 ]. To meet the demands of human population growth, it is necessary to achieve more understanding in wheat research and breeding in order to accelerate the production of wheat yield by 2050 [ 26 – 28 ]. Despite its importance, the improvement of wheat has been challenging as the researchers have to overcome the complexity of the wheat genome such as highly repetitive and large polyploid in order to get a fully sequenced reference genome [ 26 , 29 ]. Advances in next-generation sequencing (NGS) platforms and other bioinformatics tools have revealed the extensive structural rearrangements and complex gene content in wheat, which revolutionized wheat genomics with the improvement of wheat yield and its adaptation to diversed environments [ 26 , 29 ]. The NGS platforms allow the swift detection of DNA markers from the huge genome data in a short period of time. These NGS-based approaches have undoubtedly revolutionized the allele discovery and genotype-by-sequencing (GBS). By providing a high-quality reference genome of wheat in databases, it allows more sequence comparison between wheat and other species to find out more homologous gene. Moreover, the development of sequencing technologies in both high-throughput genotyping and read length, combining with biological databases, allow the rapid development of novel algorithm to complex wheat genome [ 29 , 30 ]. For instance, genome-wide association studies (GWAS) are an approach used in genome research which allows rapid screening of raw data to select specific regions with agronomic traits [ 29 , 31 ]. It allows multiple genetic variants across genome to be tested to study the genotype-phenotype association; thus, this method can be used to facilitate improvement in crop breeding via genomic selection and genetic modification [ 16 , 29 ].
Maize, a globally important crop, not only has a wide variety of uses in terms of economic impact, but can also serve as genetic model species in genotype to phenotype relationship in plant genomic studies [ 32 , 33 ]. Besides, due to its extremely high level of gene diversity, maize has high potential in the improvement of yield to meet the demands of population growth [ 33 ]. Despite the combination of economic and genomic impact, the progress in generating a whole genome sequence in maize has been a computational challenge due to the presence of tremendous structural variation (SV) in its genome [ 34 ]. The introduction of NGS techniques in several crops including maize allowed the rapid de novo genome sequencing and production of huge amount genomics and phenomics information [ 1 , 35 ]. A better integration of data within multiple genome assemblies is much needed to study the connection between phenotype and genotype in order to achieve yield and quality improvement of maize [ 35 ]. Nowadays, some user-friendly online databases such as qTeller, MaizeDIG, and MaizeMine are designed to ease the comparison and visualization of relationships between genotypes and phenotypes [ 36 ]. MaizeGDB, a model organism database for maize, provides the access of data on genes, alleles, molecular markers, metabolic pathway information, phenotypic images with description, and more which are useful for maize research [ 35 , 36 ]. MaizeMine is a data mining resource under MaizeGDB, which was designed to accelerate the genomics analysis by allowing the researchers to better script their own research data in downstream analysis [ 36 ] whereas MaizeDIG is a genotype-phenotype database which allows the users to link the association of genotype with phenotype expressed by image [ 35 , 36 ]. Cho et al. [ 35 ] reported that with the accessibility via image search tool, the relationship between a gene and its phenotype features can be visualized within image. The integration and visualization of high-quality data with these tools enables quick prioritizing phenotype of interest in crops, which play a crucial role in the improvement of plant breeding.
Bioinformatics for studying stress resistance in plants
The understanding of the stress response on plants is vital for the improvement of breeding efforts in agriculture, and to predict the fate of natural plants under abiotic change especially in the current era of continuous climate change [ 37 ]. Stress response in plants can be divided into biotic and abiotic. Biotic stress mainly refers to negative influence caused by living organism such as virus, fungi, bacteria, insects, nematodes, and weeds [ 38 ] while abiotic stress refers to factors such as extreme temperature, drought, flood, salinity, and radiation which dramatically affect the crop yield [ 37 ]. NGS technologies and other potent computational tools, which allowed sequencing of whole genome and transcriptome, have led to the extensive studies of plants towards stress response on a molecular basis [ 1 , 2 , 37 ]. The tremendous amount of plant genome data obtained from genome sequencing allows the investigation of correlations between the molecular backbone of living organism and their adaptations towards the environment [ 16 ].
Biotic and abiotic stress management
How the plants and crops respond towards stress environment is the key to ensure their growth and development, and to avoid the great crop yield penalty caused by harsh condition [ 35 , 39 ]. Therefore, the utilization of bioinformatic tools is important to study and analyse the plant transcriptome in response to biotic and abiotic stress. Besides, the application of bioinformatics tools on plants and crops genome can benefit the agricultural community by searching the desired gene among genome from different species and elucidate their function on the crops [ 35 ]. The genome databases play a crucial role in storing and mining large and complex genome sequence from the plants. Besides data storage, some genome databases are also able to perform gene expression profiling to predict the pattern of gene expressed at the level of transcript in cell or tissues. By using in silico genomic technologies, the disease resistance gene-enzyme with their respective transcription factor, which plays a role in defence mechanism against stress, are able to be identified [ 40 , 41 ]. For instance, a large-scale transcriptome sequencing of chrysanthemum plants was carried out by Xu et al. [ 40 ] to study the dehydration stress in chrysanthemum plants. An online database called Chrysanthemum Transcriptome Database ( http://www.icugi.org/chrysanthemum ) was developed to allow the storage and distribution of transcriptome sequence and its analysis result among research community [ 40 ]. With the aid of different protein databases, the biochemical pathway and kinase activity of chrysanthemum in response to dehydration stress are able to be predicted [ 40 ]. Xu et al. [ 40 ] also reported a total of 306 transcription factor and 228 protein kinase that are important upstream regulator in plants when encountered with various biotic and abiotic stresses.
Bioinformatics approaches to study resistance to plant pathogen
One of the challenges in modern agriculture to supply the nutrition’s demand along with the world population growth is the crop loss due to disease. The study of plant pathogen plays an essential role in the study of plant diseases, including pathogen identification, disease aetiology, disease resistance, and economic impact, among others [ 41 ]. Plants protect themselves through a complex defence system against variety of pathogen, including insects, bacteria, fungi, and viruses. Plant-pathogen interaction is a multicomponent system mediated by the detection of pathogen-derived molecules in the form of protein, sugar, and polysaccharide, by pattern recognition receptor (PRRs) within the plants [ 42 – 45 ]. After the recognition of enemy molecules, signal transduction is carried out accordingly and plant immune systems will respond defensively through different pathways involving different genes [ 42 ]. According to Schneider et al. [ 46 ], the development of molecular plant pathology can be broadly divided into three eras, begins with the disease physiology starting from early 1900s until 1980s [ 46 ]. In the second era of molecular plant genetic studies, one or a few genes of bacterial pathogens were focused whereas the third era of plant genomic studies began in 2000 with the sequencing of genome, and the first complete genome of bacterial pathogen, Xylella fastidiosa , was obtained [ 46 ]. The recent advance in DNA sequence technologies allow researchers to study the immune system of plants on genomic and transcriptomics level [ 1 , 41 , 42 ]. Genomics has revealed the mystery and complexity and consequently the various information about phytopathogen. A clearer picture of plant-pathogen interactions in the context of transcriptomic and proteomics can be visualized through the application of different bioinformatics tools, which in turn made feasible the engineering resistance to microbial pathogen in plant [ 43 ].
PRGdb: bioinformatics web for plant pathogen resistance gene analysis
Plants have developed a wide range of defence mechanism against different pathogen and ultimately inhibit growth and spread of pathogen [ 47 , 48 ]. Plant defence system is mediated by resistance (R) gene [ 47 ]. R gene plays an important role in defence mechanism. They encode for protein that recognizes specific avirulent (Avr) pathogen proteins and initiated the defence mechanism through one or more signal transduction pathway in a hypersensitive response (HR) [ 41 , 47 , 48 ]. However, the essential components needed for protein to exert their resistance are still unidentified [ 48 ]. With the intention to study and identify more novel R gene, high-throughput genomic experiments and plant genomic sequence are essential to explore their function and new R gene discovery [ 47 ]. In 2009, Plant Disease Resistance Gene database (PRGdb), a comprehensive bioinformatics resource across hundreds of plant species, was launched in order to facilitate the plant genome research on discovery and predict plant disease resistance gene [ 47 , 48 ]. To date, PRGdb 3.0 has been released with 153 reference resistance genes and 177,072 annotated candidate pathogen receptor genes (PRGs) [ 49 ]. This database act as an important reference site and repository to all the research studies on exploration and use of plant resistance genes [ 48 , 49 ].
Apart from resistance gene storage, this easily accessible platform also allows different tools that are essential for exploration and discovery of novel R gene. For instance, the DRAGO 2.0 tool, which was built to explore known and novel disease resistance gene, can be launched on any transcriptome or proteome to annotate and predict PRG from DNA or amino acid with high accuracy [ 49 ]. Besides, BLAST search tools available in PRGdb provide comparison of different sequences which allowed the determination of gene homology and expression analysis. Apart from the database, plant pathology field also benefited from whole genome sequence technologies. The new DNA sequencing technologies such as NGS and Sanger sequencing allowed the study of genomics, proteomics, metabolomics, and transcriptomics on both the host plant and the pathogen [ 1 ]. The phytopathogen genomes which have been sequenced are expected to provide valuable information on the molecular basis for infection of plant host and explore the potential novel virulence factors [ 1 ]. Figure 2 illustrates a brief process involved in producing stress-resistant plant using bioinformatics approach.
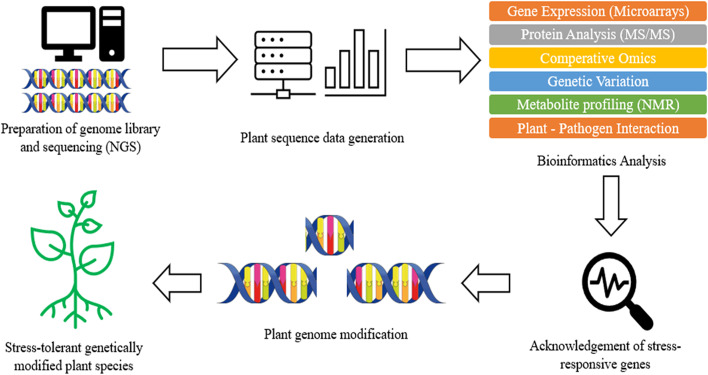
Brief process involved in producing stress-resistant plant using bioinformatics approach
Metagenomics in plant biotechnology and Cas9 modification
The effects of environment microorganisms’ community, especially soil microorganism on plants, may contribute to plant’s growth and pathogenesis. Through metagenomics approaches, the soil microorganism community that contributed to plant growth may provide a great genomic insight into physiology and pathology [ 50 – 53 ]. In metagenomics approaches, the overall genetic materials obtained from soil are sequenced and advancing to microbial community analysis via data analytics [ 53 – 55 ]. The extracted genetic materials from the soil were subjected to high-throughput metagenomics analysis via various NGS approaches such as 16S rRNA sequencing, shotgun metagenomic sequencing, MiSeq sequencing [ 54 – 56 ] for microbial species identification, functional genomics study, and structural metagenomic analysis. A NGS produces huge genomics data for each study; thus, application of bioinformatics tools would add value in the metagenomics analysis as the target genes identified could advance into elucidation of plant growth, plant disease, soil contamination, and microbial taxonomy [ 52 ]. For example, the use of UNITE ( https://unite.ut.ee/ ) for fungi identification [ 57 ], SILVA ( https://www.arb-silva.de/ ) for 16S rRNA [ 58 ], and MGnify ( https://www.ebi.ac.uk/metagenomics/ ) possesses metagenomics data of microbiome [ 59 ]. These databases allow the researchers to retrieve and analyse the relevant metagenomic sequenced data for a specific study.
Since metagenomics analysis provides the greater output on plant-microbe interaction, the genes that are responsible for plant immunity may play a crucial role in protecting against disease-causing microorganism [ 60 , 61 ]. With the emergence of Clustered Regularly Interspaced Short Palindrome Repeats (CRISPR) gene editing technique, Cas9 modification could produce a better plant trait and disease-resistant plant [ 62 , 63 ]. The CRISPR/Cas9 system is employed in studying the functional genomics in plants in relation to plant-microbe interaction. CRISPR/Cas9 system facilitated the gene editing by creating a mutant through double-stranded break forming a targeted gene mutation and followed by genome repair [ 63 – 65 ]. The CRISPR/Cas9 modification on OsSWEET14 genes protects the Super Basmati Rice from bacterial blight causes by Xanthomonas oryzae pv. oryzae [ 66 ]. Gene editing to knockout OsMPK5 and OsERF922 genes in rice protects against Magnaporthe grisea and Magnaporthe oryzae , respectively [ 67 – 69 ]. Besides that, Cas9 modification on Cs WRKY22 and TcNPR3 increased host defence immunity through regulating salicylic acid in Citrus sinensis and Theobroma cacao , respectively [ 70 , 71 ]. Thus, CRISPR/Cas9 modification could be one of important science advancements to validate the metagenomics analysis on plant-microbe interaction.
Current challenges of bioinformatics applications in plant biotechnology
Despite the beneficial prospect of the bioinformatics applied in plant biotechnology, there are many challenges and limitations must be addressed in order to fully utilize their potentials [ 1 ]. Along with the rapid growth in plant genome data mining and database development, there are a few challenges faced by bioinformaticians and scientists which can be divided into number of areas as mentioned in the subsections below.
Bioinformatic data management and organization and synchronize update resources
Since the introduction of the next-generation sequencing (NGS), which is commercially available in 2004, enormous amount of data has been generated in plant genome research. Thousands of Gb of plants sequences are deposited in various public databases monthly [ 1 , 72 , 73 ]. Moreover, the constantly sequenced and re-sequenced of the plant genome has developed a vast amount of new genome sequence in all public databases. The increase in sequenced plant genome driven by technological improvement has led to a problem that arises along with the storage and update of a large amount of data [ 72 , 74 ]. The update process should occur in all the comparative databases, not just solely individual genome database [ 72 ]. With this, the synchronized update of genome data resources among different plant genomic platform is able to provide a strong, updated, reliable database community that all the plant researchers can rely on [ 72 ].
Complexity of plant genetic content
Other than the tremendous amount of genome sequence generated, the complexity of the plant genetic content is also a challenging issue faced by plant research community. Even though the arrival of next-generation sequencing technologies has allowed the rapid DNA sequencing for non-model or orphan plant species, the sequencing pace for plants is far from that of animal and microorganism [ 74 ]. The main factor which contributes to this situation is because sometimes the plant genome can be nearly hundred times larger than the currently sequenced animal and microorganism genome [ 73 ]. Needless to say, some of the plant genome even can have polyploidy, a duplication of an entire genome, which is estimated to occur in 80% of the plant species [ 73 , 75 ]. According to Schatz et al., the genome assembly in the case of large size plant genome with abundance of repetitive sequence can be metaphorically described as build-up of a large puzzle consisting of blue sky separated by nearly indistinguishable white clouds of small gene [ 73 ]. The particular reason for this is mainly because the sequence length in NGS is relatively shorter than in Sanger sequencing and required dedicated assembly algorithm [ 74 ]. Therefore, most plant genomes sequenced by NGS can only be used for establishing gene catalogues, interpreting the repeat content, glimpsing evolutionary mechanism, and performing on comparative genomics in early study [ 74 ].
Advance in sequencing technologies
There are two basic approaches to genome assembly, i.e. comparative genome assembly and de novo genome assembly [ 75 ]. It is important to distinguish between these two different approaches. Comparative is a reference-guided method which use a genome or transcriptome, or both, for guidance, whereas de novo assembly refers to reconstruction of a genome from organisms that have not been sequenced before [ 74 , 75 ]. Table 2 compares some of the available assembly and NGS technology available for genome sequencing. However, these two approaches are not completely exclusive due to a lack of bioinformatic tools designed to cope with the unique and challenging features of plant genomes [ 74 , 75 ]. One of the biggest challenges in the development of bioinformatic software is the algorithm development [ 76 ]. As is known, all the programmes or software in bioinformatic are very computationally intensive. As most of the assemblies available now solely rely on single assembly, a development in better algorithm in terms of resource requirement is essential for combining different assemblers by using a different underlying algorithm in order to give a more credible final assembly [ 74 , 76 ].
Comparison between next-generation sequencing technologies
Database accessibility
To date, there are about 374,000 known plant species in the world [ 77 ]. The first full plant genome sequencing was completed on A rabidopsis thaliana through Sanger sequencing methods in 2000 [ 78 ]. Although introduction of molecular biology decades ago may have facilitated the species identification, obtaining the full plant genomic data remains challenging due to the genome complexity. The development of NGS platform may foster the plant genome sequencing, yet there are limited sequenced datasets reposited to the database. To date, there are only 29 plant genome databases accessible in PlantGDB genome browser allowing researchers to retrieve the information about gene structure, matched GSS contigs, similar protein, spliced alignments EST, etc. Besides, the PlaD database ( http://systbio.cau.edu.cn/plad/index.php ) that focuses on the microarray data of the plants developed by China Agricultural University comprises transcriptomic database for plant defence against pathogen. However, it is limited to Arabidopsis , rice, maize, and wheat [ 79 ]. The Plant Omics Data Center ( http://plantomics.mind.meiji.ac.jp/podc/ ) is another publicly available web-based plant database featuring omics data for co-expressed profile, regulatory network, and plant ontology information [ 80 ]. Although curated omics datasets could be retrieved from PODC, information are restricted for certain plants and crops such as Arabidopsis , tobacco, earthmoss, barrelclover, soybean, potato, rice, tomato, grape, maize, and sorghum. Furthermore, all these publicly available databases require constant updating with new released data or resequencing data so that the researcher could obtain the most updated version of genome datasets for their research.
The application of bioinformatics in plant biotechnology represents a fundamental shift in the way scientists study living organisms. Bioinformatics play a significant role in the development of agriculture sector as it helps to study the stress resistance and plant pathogen, which are critical in advancing crop breeding [ 75 ]. NGS and other sequencing technologies will make more plant genome data accessible in all public databases and enable the identification of genomic variants and prediction of protein structure and function [ 75 , 76 ]. Moreover, GWAS, which allows the identification of loci and allelic variation related to valuable traits, eased the crop modification and improvement [ 74 ]. In brief, the advance in bioinformatics application in plant biotechnology enables researchers to achieve fundamental and systematic understanding of economically important plant. However, despite all these exciting achievement by the application of bioinformatic on plant biotechnology, it is still a long way from automated full genome sequencing and assembly at a low cost [ 76 ]. There is a critical need for effective bioinformatic tools which are able to provide longer reads with unbiased coverage in order to overcome the complexity of the plant’s genome. To achieve this, an enhanced algorithm development is essential to enable data mining and analysis, comparison, and so on. Therefore, bioinformaticians and experts with mathematical and programming skills will play an important role in bringing fresh approaches and knowledge into bioinformatics, not only for the advancement in plant biotechnology and agriculture sector, but the future of humanity as well.
Acknowledgements
The authors wish to thank Prof. Hoe I. Ling of Columbia University (New York, USA) for his editorial input and proofread the manuscript.
Abbreviations
Genome-wide association studies
Next-generation sequencing
Plant Disease Resistance Gene database
RNA sequencing
Single-nucleotide polymorphism
Authors’ contributions
YCT designed the content and was a major contributor in writing the manuscript. AUK and YPW edited the manuscript. APKL designed and edited the manuscript. All authors read and approved the final manuscript.
Not applicable.
Availability of data and materials
Declarations, ethics approval and consent to participate, consent for publication, competing interests.
The authors declare that they have no competing interests.
Publisher’s Note
Springer Nature remains neutral with regard to jurisdictional claims in published maps and institutional affiliations.
- 1. Gomez-Casati DF, Busi MV, Barchiesi J, Peralta DA, Hedin N, Bhadauria V. Applications of bioinformatics to plant biotechnology. Curr Issues Mol Biol. 2018;27:89–104. doi: 10.21775/cimb.027.089. [ DOI ] [ PubMed ] [ Google Scholar ]
- 2. Zhang SY, Liu SL. Bioinformatics. In: Maloy S, Hughes K, editors. Brenner’s Encyclopedia of Genetics. 2. London: Academic Press; 2013. [ Google Scholar ]
- 3. Tiwari A, Singh P, Kumawat S. Applications of bioinformatics in plant breeding system. Int J Curr Microbial App Sci. 2020;11:2825–2831. [ Google Scholar ]
- 4. Rhee SY, Dickerson J, Xu D. Bioinformatics and its applications in plant biology. Annu Rev Plant Biol. 2006;57:335–360. doi: 10.1146/annurev.arplant.56.032604.144103. [ DOI ] [ PubMed ] [ Google Scholar ]
- 5. Normand EA, Van den Veyyer IB. Next-generation sequencing for gene panels and clinical exomes. In: Leung PCK, Qiao J, editors. Human Reproductive and Prenatal Genetics. 1. London: Academic Press; 2019. [ Google Scholar ]
- 6. Blätke MA, Szymanski JJ, Gladilin E, Scholz U, Beier S. Editorial: advances in applied bioinformatics in crops. Front Plant Sci. 2021;12:640394. doi: 10.3389/fpls.2021.640394. [ DOI ] [ PMC free article ] [ PubMed ] [ Google Scholar ]
- 7. Kushwaha UKS, Deo I, Jaiswal JP, Prasad B. Role of bioinformatics in crop improvement. Glob J Sci Front Res D Agric Vet. 2017;17(1):13–23. [ Google Scholar ]
- 8. Caligari PDS, Brown J. Plant Breeding, Practice. In: Thomas B, Murray BG, Murphy DJ, editors. Encyclopedia of Applied Plant Sciences. 2. London: Academic Press; 2017. [ Google Scholar ]
- 9. Yu J, Jung S, Cheng CH, Lee T, Zheng P, Buble K, et al. CottonGen: the community database for cotton genomics, genetics, and breeding research. Plants. 2021;10(12):2805. doi: 10.3390/plants10122805. [ DOI ] [ PMC free article ] [ PubMed ] [ Google Scholar ]
- 10. Sayers EW, Bolton EE, Brister JR, Canese K, Chan J, Comeau DC, et al. Database resources of the national center for biotechnology information. Nucleic Acids Res. 2022;50(D1):D20–D26. doi: 10.1093/nar/gkab1112. [ DOI ] [ PMC free article ] [ PubMed ] [ Google Scholar ]
- 11. Howe KL, Contreras-Moreira B, De Silva N, Maslen G, Akanni W, Allen J, et al. Ensembl Genomes 2020 – enabling non-vertebrate genomic research. Nucleic Acids Res. 2019;48(D1):D689–D695. doi: 10.1093/nar/gkz890. [ DOI ] [ PMC free article ] [ PubMed ] [ Google Scholar ]
- 12. Bolser D, Staines DM, Pritchard E, Kersey P. Ensembl plants: integrating tools for visualizing, mining, and analyzing plant genomics data. In: Edwards D, editor. Plant Bioinformatics. Methods in Molecular Biology, vol 1374. Humana Press; 2016. [ DOI ] [ PubMed ] [ Google Scholar ]
- 13. Jhansi Rani S, Usha R. Transgenic plants: Types, benefits, public concerns and future. J Pharm Res. 2013;6(8):879–883. doi: 10.1016/j.jopr.2013.08.008. [ DOI ] [ Google Scholar ]
- 14. Barragán-Ocaña A, Reyes-Ruiz G, Olmos-Peña S, Gómez-Viquez H. Transgenic crops: trends and dynamics in the world and in Latin America. Transgenic Res. 2019;28(3-4):391–399. doi: 10.1007/s11248-019-00123-8. [ DOI ] [ PubMed ] [ Google Scholar ]
- 15. Platten JD, Cobb JN, Zantua RE. Criteria for evaluating molecular markers: Comprehensive quality metrics to improve marker-assisted selection. PLoS One. 2019;14(1):e0210529. doi: 10.1371/journal.pone.0210529. [ DOI ] [ PMC free article ] [ PubMed ] [ Google Scholar ]
- 16. Filho HA, Machicao J, Bruno OM. A hierarchical model of metabolic machinery based on the kcore decomposition of plant metabolic networks. PLoS One. 2018;13(5):e0195843. doi: 10.1371/journal.pone.0195843. [ DOI ] [ PMC free article ] [ PubMed ] [ Google Scholar ]
- 17. Mammadov J, Aggarwal R, Buyyarapu R, Kumpatla S. SNP markers and their impact on plant breeding. Int J Plant Genomics. 2012;728398:1–11. doi: 10.1155/2012/728398. [ DOI ] [ PMC free article ] [ PubMed ] [ Google Scholar ]
- 18. Hoskins RA, Phan AC, Naeemuddin M, Mapa FA, Ruddy DA, Ryan JJ, et al. Single nucleotide polymorphism markers for genetics mapping in Drosophila melanogaster. Genome Res. 2001;11(6):1100–1113. doi: 10.1101/gr.gr-1780r. [ DOI ] [ PMC free article ] [ PubMed ] [ Google Scholar ]
- 19. Edwards D, Batley J. Plant genome sequencing: applications for crop improvement. Plant Biotechnol J. 2010;8(1):2–9. doi: 10.1111/j.1467-7652.2009.00459.x. [ DOI ] [ PubMed ] [ Google Scholar ]
- 20. Tang G, Qin J, Dolnikowski GG, Russell RM, Grusak MA. Golden Rice is an effective source of vitamin A. Am J Clin Nutr. 2009;89(6):1776–1783. doi: 10.3945/ajcn.2008.27119. [ DOI ] [ PMC free article ] [ PubMed ] [ Google Scholar ]
- 21. Yu J, Hu S, Wang J, Wong GKS, Li S, Liu B, et al. A draft sequence of the rice genome (Oryza sativa L. ssp. Indica) Science. 2002;296(5565):79–92. doi: 10.1126/science.1068037. [ DOI ] [ PubMed ] [ Google Scholar ]
- 22. Song S, Tian D, Zhang Z, Hu S, Yu J. Rice genomics: over the past two decades and into the future. Genomics Proteomics Bioinformatics. 2018;16(6):397–404. doi: 10.1016/j.gpb.2019.01.001. [ DOI ] [ PMC free article ] [ PubMed ] [ Google Scholar ]
- 23. Jackson SA (2016) Rice: The First Crop Genome. Rice. 9(14). 10.1186/s12284-016-0087-4 [ DOI ] [ PMC free article ] [ PubMed ]
- 24. Jain R, Jenkins J, Shu S, Chern M, Martin JA, Copetti D et al (2019) Genome sequence of the model rice variety KitaakeX. BMC Genomics 20(905). 10.1186/s12864-019-6262-4 [ DOI ] [ PMC free article ] [ PubMed ]
- 25. Vassilev D, Leunissen J, Atanassov A, Nenov A, Dimov G. Application of bioinformatics in plant breeding. Biotechnol Biotechnol Equip. 2005;19(sup3):139–152. doi: 10.1080/13102818.2005.10817293. [ DOI ] [ Google Scholar ]
- 26. Walkowiak S, Gao L, Monat C, Haberer G, Kassa MT, Brinton J, et al. Multiple wheat genomes reveal global variation in modern breeding. Nature. 2020;588(7837):277–283. doi: 10.1038/s41586-020-2961-x. [ DOI ] [ PMC free article ] [ PubMed ] [ Google Scholar ]
- 27. Appels R, Eversole K, Stein N, Feuillet C, Keller B, Rogers J et al (2018) Shifting the limits in wheat research and breeding using a fully annotated reference genome. Science. 361(6403). 10.1126/science.aar7191 [ DOI ] [ PubMed ]
- 28. Gill BS, Appels R, Borta-Oberholster AM, Buell CR, Bennetzen JL, Chalhoub B, et al. A workshop report on wheat genome sequencing: International Genome Research on Wheat Consortium. Genetics. 2004;168(2):1087–1096. doi: 10.1534/genetics.104.034769. [ DOI ] [ PMC free article ] [ PubMed ] [ Google Scholar ]
- 29. Babu P, Baranwal DK, Harikrishna PD, Bharti H, Joshi P, et al. Application of genomics tools in wheat breeding to attain durable rust resistance. Front Plant Sci. 2020;11:567147. doi: 10.3389/fpls.2020.567147. [ DOI ] [ PMC free article ] [ PubMed ] [ Google Scholar ]
- 30. Guan J, Garcia DF, Zhou Y, Appels R, Li A, Mao L. The battle to sequence the bread wheat genome: a tale of the three kingdoms. Genomics Proteomics Bioinformatics. 2020;18(3):221–229. doi: 10.1016/j.gpb.2019.09.005. [ DOI ] [ PMC free article ] [ PubMed ] [ Google Scholar ]
- 31. Bolser D, Staines DM, Pritchard E, Kersey P. Ensembl plants: integrating tools for visualizing, mining and analyzing plant genomics data. Methods Mol Biol. 2016;1374:115–140. doi: 10.1007/978-1-4939-3167-5_6. [ DOI ] [ PubMed ] [ Google Scholar ]
- 32. Haberer G, Young S, Bharti AK, Gundlach H, Raymond C, Fuks G, et al. Structure and architecture of the maize genome. Plant Physiol. 2005;139(4):1612–1624. doi: 10.1104/pp.105.068718. [ DOI ] [ PMC free article ] [ PubMed ] [ Google Scholar ]
- 33. Li C, Song W, Luo Y, Gao S, Zhang R, Shi Z, et al. The HuangZaoSi maize genome provides insights into genomic variation and improvement history of maize. Mol Plant. 2019;12(3):402–409. doi: 10.1016/j.molp.2019.02.009. [ DOI ] [ PubMed ] [ Google Scholar ]
- 34. Lu F, Romay MC, Glaubitz JC, Bradbury PJ, Elshire RJ, Wang T, et al. High-resolution genetic mapping of maize pan-genome sequence anchors. Nat Commun. 2015;6:6914. doi: 10.1038/ncomms7914. [ DOI ] [ PMC free article ] [ PubMed ] [ Google Scholar ]
- 35. Cho KT, Portwood JL, Gardiner JM, Harper LC, Lawrence-Dill CJ, Friedberg I, et al. MaizeDIG: maize database of images and genomes. Front Plant Sci. 2019;10:1050. doi: 10.3389/fpls.2019.01050. [ DOI ] [ PMC free article ] [ PubMed ] [ Google Scholar ]
- 36. Portwood JL, Woodhouse MR, Cannon EK, Gardiner JM, Harper LC, Schaeffer ML, et al. MaizeGDB 2018: the maize multi-genome genetics and genomics database. Nucleic Acids Res. 2018;47(D1):D1146–D1154. doi: 10.1093/nar/gky1046. [ DOI ] [ PMC free article ] [ PubMed ] [ Google Scholar ]
- 37. Ambrosino L, Colantuono C, Diretto G, Fiore A, Chiusano ML. Bioinformatics resources for plant abiotic stress responses: state of the art and opportunities in the fast evolving -omics era. Plants. 2020;9(5):591. doi: 10.3390/plants9050591. [ DOI ] [ PMC free article ] [ PubMed ] [ Google Scholar ]
- 38. Singla J, Krattinger SG (2016) Biotic stress resistance genes in wheat. Reference Module in Food Science. 10.1016/B978-0-08-100596-5.00229-8
- 39. Costa MCD, Farrant JM. Plant resistance to abiotic stresses. Plants (Basel) 2019;8(12):553. doi: 10.3390/plants8120553. [ DOI ] [ PMC free article ] [ PubMed ] [ Google Scholar ]
- 40. Xu Y, Gao S, Yang Y, Huang M, Cheng L, Wei Q, et al. Transcriptome sequencing and whole genome expression profiling of chrysanthemum under dehydration stress. BMC Genomics. 2013;14:662. doi: 10.1186/1471-2164-14-662. [ DOI ] [ PMC free article ] [ PubMed ] [ Google Scholar ]
- 41. Nishad R, Ahmed T, Rahman VJ, Kareem A. Modulation of plant defense system in response to microbial interactions. Front Microbiol. 2020;11:1298. doi: 10.3389/fmicb.2020.01298. [ DOI ] [ PMC free article ] [ PubMed ] [ Google Scholar ]
- 42. Andersen EJ, Ali S, Byamukama E, Yen Y, Nepal MP. Disease resistance mechanisms in plants. Genes (Basel) 2018;9(7):339. doi: 10.3390/genes9070339. [ DOI ] [ PMC free article ] [ PubMed ] [ Google Scholar ]
- 43. Dong OX, Ronald PC. Genetic engineering for disease resistance in plants: recent progress and future perspectives. Plant Physiol. 2019;180(1):26–38. doi: 10.1104/pp.18.01224. [ DOI ] [ PMC free article ] [ PubMed ] [ Google Scholar ]
- 44. Abdulkhair WM, Alghuthaymi MA. Plant pathogens. In: Rigobelo EC, editor. Plant Growth, 1st edn. InTechOpen. 2016. [ Google Scholar ]
- 45. Gupta R, Lee SE, Agrawal GK, Rakwal R, Sangryeol P, Wang Y, et al. Understanding the plant-pathogen interactions in the context of proteomics-generated apoplastic proteins inventory. Front Plant Sci. 2015;6:352. doi: 10.3389/fpls.2015.00352. [ DOI ] [ PMC free article ] [ PubMed ] [ Google Scholar ]
- 46. Schneider DJ, Collmer A. Studying plant-pathogen interactions in the genomics era: beyond Molecular Koch’s postulates to systems biology. Annu Rev Phytopathol. 2010;48:457–479. doi: 10.1146/annurev-phyto-073009-114411. [ DOI ] [ PubMed ] [ Google Scholar ]
- 47. Sanseverino W, Hermoso A, D’Alessandro R, Vlasova A, Andolfo G, Frusciante L, et al. PRGdb 2.0: towards a community-based database model for the analysis of R-genes in plants. Nucleic Acids Res. 2013;41(Database Issue):D1167–D1171. doi: 10.1093/nar/gks1183. [ DOI ] [ PMC free article ] [ PubMed ] [ Google Scholar ]
- 48. Sanseverino W, Roma G, Simone MD, Faino L, Melito S, Stupka E, et al. PRGdb: a bioinformatics platform for plant resistance gene analysis. Nucleic Acids Res. 2010;38(Database Issue):D814–D821. doi: 10.1093/nar/gkp978. [ DOI ] [ PMC free article ] [ PubMed ] [ Google Scholar ]
- 49. Osuna-Cruz CM, Paytuvi-Gallart A, Donato AD, Sundesha V, Andolfo G, Cigliano RA, et al. PRGdb 3.0: a comprehensive platform for prediction and analysis of plant disease resistance genes. Nucleic Acids Res. 2018;46(D1):D1197–D1201. doi: 10.1093/nar/gkx1119. [ DOI ] [ PMC free article ] [ PubMed ] [ Google Scholar ]
- 50. Hily JM, Demanèche S, Poulicard N, Tannières M, Djennane S, Beuve M, et al. Metagenomic-based impact study of transgenic grapevine rootstock on its associated virome and soil bacteriome. Plant Biotechnol J. 2018;16(1):208–220. doi: 10.1111/pbi.12761. [ DOI ] [ PMC free article ] [ PubMed ] [ Google Scholar ]
- 51. Fadiji AE, Babalola OO. Metagenomics methods for the study of plant-associated microbial communities: a review. J Microbiol Methods. 2020;70:105860. doi: 10.1016/j.mimet.2020.105860. [ DOI ] [ PubMed ] [ Google Scholar ]
- 52. Piombo E, Abdelfattah A, Droby S, Wisniewski M, Spadaro D, Schena L. Metagenomics approaches for the detection and surveillance of emerging and recurrent plant pathogens. Microorganisms. 2021;9(1):188. doi: 10.3390/microorganisms9010188. [ DOI ] [ PMC free article ] [ PubMed ] [ Google Scholar ]
- 53. Chaudhary P, Khati P, Chaudhary A, Maithani D, Kumar G, Sharma A. Cultivable and metagenomic approach to study the combined impact of nanogypsum and Pseudomonas taiwanensis on maize plant health and its rhizospheric microbiome. PLoS One. 2021;16(4):e0250574. doi: 10.1371/journal.pone.0250574. [ DOI ] [ PMC free article ] [ PubMed ] [ Google Scholar ]
- 54. Chukwuneme CF, Ayangbenro AS, Babalola OO. Metagenomic analyses of plant growth-promoting and carbon-cycling genes in maize rhizosphere soils with distinct land-use and management histories. Genes (Basel) 2021;12(9):1431. doi: 10.3390/genes12091431. [ DOI ] [ PMC free article ] [ PubMed ] [ Google Scholar ]
- 55. Zhao J, Ma J, Yang Y, Yu H, Zhang S, Chen F. Response of soil microbial community to vegetation reconstruction modes in mining areas of the Loess Plateau, China. Front Microbiol. 2021;12:714967. doi: 10.3389/fmicb.2021.714967. [ DOI ] [ PMC free article ] [ PubMed ] [ Google Scholar ]
- 56. Babalola OO, Fadiji AE, Ayangbenro AS (2020) Shotgun metagenomic data of root endophytic microbiome of maize ( Zea mays L.). Data Brief 31(105893). 10.1016/j.dib.2020.105893 [ DOI ] [ PMC free article ] [ PubMed ]
- 57. Nilsson RH, Larsson KH, Taylor AFS, Bengtsson-Palme J, Jeppesen TS, Schigel D, et al. The UNITE database for molecular identification of fungi: handling dark taxa and parallel taxonomic classifications. Nucleic Acids Res. 2019;47(D1):D259–D264. doi: 10.1093/nar/gky1022. [ DOI ] [ PMC free article ] [ PubMed ] [ Google Scholar ]
- 58. Quast C, Pruesse E, Yilmaz P, et al. The SILVA ribosomal RNA gene database project: improved data processing and web-based tools. Nucleic Acids Res. 2013;41(Database issue):D590–D596. doi: 10.1093/nar/gks1219. [ DOI ] [ PMC free article ] [ PubMed ] [ Google Scholar ]
- 59. Mitchell AL, Almeida A, Beracochea M, Boland M, Burgin J, Cochrane G, et al. MGnify: the microbiome analysis resource in 2020. Nucleic Acids Res. 2020;48(D1):D570–D578. doi: 10.1093/nar/gkz1035. [ DOI ] [ PMC free article ] [ PubMed ] [ Google Scholar ]
- 60. Musidlak O, Buchwald W, Nawrot R. Plant defense responses against viral and bacterial pathogen infections. Focus on RNA-binding proteins (RBPs) Herba Polonica. 2014;60:60–73. doi: 10.1515/hepo-2015-0005. [ DOI ] [ Google Scholar ]
- 61. Silva MS, Arraes FBM, Campos MDA, Grossi-de-Sa M, Fernandez D, Cândido EDS, et al. Review: potential biotechnological assets related to plant immunity modulation applicable in engineering disease-resistant crops. Plant Sci. 2018;270:72–84. doi: 10.1016/j.plantsci.2018.02.013. [ DOI ] [ PubMed ] [ Google Scholar ]
- 62. Feng Z, Zhang B, Ding W, Liu X, Yang DL, Wei P, et al. Efficient genome editing in plants using a CRISPR/Cas system. Cell Res. 2013;23(10):1229–1232. doi: 10.1038/cr.2013.114. [ DOI ] [ PMC free article ] [ PubMed ] [ Google Scholar ]
- 63. Wada N, Ueta R, Osakabe Y, Osakabe K. Precision genome editing in plants: state-of-the-art in CRISPR/Cas9-based genome engineering. BMC Plant Biol. 2020;20:234. doi: 10.1186/s12870-020-02385-5. [ DOI ] [ PMC free article ] [ PubMed ] [ Google Scholar ]
- 64. Nekrasov V, Staskawicz B, Weigel D, Jones JD, Kamoun S. Targeted mutagenesis in the model plant Nicotiana benthamiana using Cas9 RNA-guided endonuclease. Nat Biotechnol. 2013;31(8):691–693. doi: 10.1038/nbt.2655. [ DOI ] [ PubMed ] [ Google Scholar ]
- 65. Langner T, Kamoun S, Belhaj K. CRISPR crops: plant genome editing toward disease resistance. Annu Rev Phytopathol. 2018;56:479–512. doi: 10.1146/annurev-phyto-080417-050158. [ DOI ] [ PubMed ] [ Google Scholar ]
- 66. Zafar K, Khan MZ, Amin I, Mukhtar Z, Yasmin S, Arif M, et al. Precise CRISPR-Cas9 mediated genome editing in super basmati rice for resistance against bacterial blight by targeting the major susceptibility gene. Front Plant Sci. 2020;11:575. doi: 10.3389/fpls.2020.00575. [ DOI ] [ PMC free article ] [ PubMed ] [ Google Scholar ]
- 67. Xie K, Yang Y. RNA-guided genome editing in plants using a CRISPR-Cas system. Mol Plant. 2013;6(6):1975–1983. doi: 10.1093/mp/sst119. [ DOI ] [ PubMed ] [ Google Scholar ]
- 68. Wang F, Wang C, Liu P, Lei C, Hao W, Gao Y, et al. Enhanced rice blast resistance by CRISPR/Cas9-targeted mutagenesis of the ERF transcription factor gene OsERF922. PLoS One. 2016;11(4):e0154027. doi: 10.1371/journal.pone.0154027. [ DOI ] [ PMC free article ] [ PubMed ] [ Google Scholar ]
- 69. Oliva R, Ji C, Atienza-Grande G, Huguet-Tapia JC, Perez-Quintero A, Li T, et al. Broad-spectrum resistance to bacterial blight in rice using genome editing. Nat Biotechnol. 2019;37(11):1344–1350. doi: 10.1038/s41587-019-0267-z. [ DOI ] [ PMC free article ] [ PubMed ] [ Google Scholar ]
- 70. Wang L, Chen S, Peng A, Xie Z, He Y, Zou X. CRISPR/CAS9 -mediated editing of CsWRKY22 reduces susceptibility to Xanthomonas citri subsp. citri in Wanjincheng orange (Citrus sinensis (L.) Osbeck) Plant Biotechnol Rep. 2019;13(5):501–510. doi: 10.1007/s11816-019-00556-x. [ DOI ] [ Google Scholar ]
- 71. Fister AS, Landherr L, Maximova SN, Guiltinan MJ. Transient expression of CRISPR/Cas9 machinery targeting TcNPR3 Enhances defense response in theobroma cacao. Front Plant Sci. 2018;9:268. doi: 10.3389/fpls.2018.00268. [ DOI ] [ PMC free article ] [ PubMed ] [ Google Scholar ]
- 72. Ong Q, Nguyen P, Thao NP, Le L. Bioinformatics approach in plant genomic research. Curr Genomics. 2016;17(4):368–378. doi: 10.2174/1389202917666160331202956. [ DOI ] [ PMC free article ] [ PubMed ] [ Google Scholar ]
- 73. Schatz MC, Witkowski J, McCombie WR. Current challenges in de novo plant genome sequencing and assembly. Genome Biol. 2012;13(4):243. doi: 10.1186/gb-2012-13-4-243. [ DOI ] [ PMC free article ] [ PubMed ] [ Google Scholar ]
- 74. Claros MG, Bautista R, Guerrero-Fernández D, Benzerki H, Seoane P, Fernández-Pozo N. Why assembling plant genome sequences is so challenging. Biology (Basel) 2012;1(2):439–459. doi: 10.3390/biology1020439. [ DOI ] [ PMC free article ] [ PubMed ] [ Google Scholar ]
- 75. Kyriakidou M, Tai HH, Anglin NL, Ellis D, Strömvik MV. Current strategies of polyploid plant genome sequence assembly. Front Plant Sci. 2018;9:1660. doi: 10.3389/fpls.2018.01660. [ DOI ] [ PMC free article ] [ PubMed ] [ Google Scholar ]
- 76. Mathur M. Bioinformatics challenges: a review. Int J Adv Sci Res. 2018;3(6):29–33. [ Google Scholar ]
- 77. Fazan L, Song YG, Kozlowski G. The woody planet: from past triumph to manmade decline. Plants (Basel) 2020;9(11):1593. doi: 10.3390/plants9111593. [ DOI ] [ PMC free article ] [ PubMed ] [ Google Scholar ]
- 78. Arabidopsis Genome Initiative Analysis of the genome sequence of the flowering plant Arabidopsis thaliana. Nature. 2000;408(6814):796–815. doi: 10.1038/35048692. [ DOI ] [ PubMed ] [ Google Scholar ]
- 79. Qi H, Jiang Z, Zhang K, Yang S, He F, Zhang Z. PlaD: a transcriptomics database for plant defense responses to pathogens, providing new insights into plant immune system. Genomics Proteomics Bioinformatics. 2018;16(4):283–293. doi: 10.1016/j.gpb.2018.08.002. [ DOI ] [ PMC free article ] [ PubMed ] [ Google Scholar ]
- 80. Ohyanagi H, Takano T, Terashima S, Kobayashi M, Kanno M, Morimoto K, et al. Plant Omics Data Center: an integrated web repository for interspecies gene expression networks with NLP-based curation. Plant Cell Physiol. 2015;56(1):e9. doi: 10.1093/pcp/pcu188. [ DOI ] [ PMC free article ] [ PubMed ] [ Google Scholar ]
Associated Data
This section collects any data citations, data availability statements, or supplementary materials included in this article.
Data Availability Statement
- View on publisher site
- PDF (1.3 MB)
- Collections
Similar articles
Cited by other articles, links to ncbi databases.
- Download .nbib .nbib
- Format: AMA APA MLA NLM
Add to Collections
Recent Advances in Plant Biotechnology
- © 2009
- Ara Kirakosyan 0 ,
- Peter B. Kaufman 1
University of Michigan, Ann Arbor, U.S.A.
You can also search for this author in PubMed Google Scholar
- Presents a full overview of plant biotechnology from the history to applications
- Approach includes associated risks and the effects of plant biotechnology on global warming, alternative energy initiatives, food production, and medicine
- Includes supplementary material: sn.pub/extras
33k Accesses
81 Citations
4 Altmetric
This is a preview of subscription content, log in via an institution to check access.
Access this book
Subscribe and save.
- Get 10 units per month
- Download Article/Chapter or eBook
- 1 Unit = 1 Article or 1 Chapter
- Cancel anytime
- Available as EPUB and PDF
- Read on any device
- Instant download
- Own it forever
- Compact, lightweight edition
- Dispatched in 3 to 5 business days
- Free shipping worldwide - see info
- Durable hardcover edition
Tax calculation will be finalised at checkout
Other ways to access
Licence this eBook for your library
Institutional subscriptions
About this book
Similar content being viewed by others.
History of Plant Biotechnology Development
Creating Products and Services in Plant Biotechnology
- agriculture
- alternative energy
- bioremediation
- biotechnology
- genetically modified plants
- herbal medicine
- herbal products
- plant biotechnology
- transgenic plants
Table of contents (16 chapters)
Front matter, plant biotechnology from inception to the present, overview of plant biotechnology from its early roots to the present.
- Ara Kirakosyan, Peter B. Kaufman, Leland J. Cseke
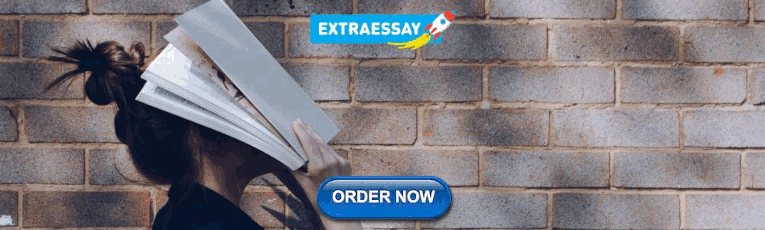
The Use of Plant Cell Biotechnology for the Production of Phytochemicals
- Ara Kirakosyan, Leland J. Cseke, Peter B. Kaufman
Molecular Farming of Antibodies in Plants
- Rainer Fischer, Stefan Schillberg, Richard M. Twyman
Use of Cyanobacterial Proteins to Engineer New Crops
- Matias D. Zurbriggen, Néstor Carrillo, Mohammad-Reza Hajirezaei
Molecular Biology of Secondary Metabolism: Case Study for Glycyrrhiza Plants
- Hiroaki Hayashi
Applications of Plant Biotechnology in Agriculture and Industry
New developments in agricultural and industrial plant biotechnology, phytoremediation: the wave of the future.
- Jerry S. Succuro, Steven S. McDonald, Casey R. Lu
Biotechnology of the Rhizosphere
- Beatriz Ramos Solano, Jorge Barriuso Maicas, Javier Gutierrez Mañero
Plants as Sources of Energy
- Leland J. Cseke, Gopi K. Podila, Ara Kirakosyan, Peter B. Kaufman
Use of Plant Secondary Metabolites in Medicine and Nutrition
Interactions of bioactive plant metabolites: synergism, antagonism, and additivity.
- John Boik, Ara Kirakosyan, Peter B. Kaufman, E. Mitchell Seymour, Kevin Spelman
The Use of Selected Medicinal Herbs for Chemoprevention and Treatment of Cancer, Parkinson’s Disease, Heart Disease, and Depression
- Maureen McKenzie, Carl Li, Peter B. Kaufman, E. Mitchell Seymour, Ara Kirakosyan
Regulating Phytonutrient Levels in Plants – Toward Modification of Plant Metabolism for Human Health
Risks and benefits associated with plant biotechnology, risks and benefits associated with genetically modified (gm) plants.
- Peter B. Kaufman, Soo Chul Chang, Ara Kirakosyan
Risks Involved in the Use of Herbal Products
- Peter B. Kaufman, Maureen McKenzie, Ara Kirakosyan
Risks Associated with Overcollection of Medicinal Plants in Natural Habitats
- Maureen McKenzie, Ara Kirakosyan, Peter B. Kaufman
Authors and Affiliations
Ara Kirakosyan, Peter B. Kaufman
About the authors
Bibliographic information.
Book Title : Recent Advances in Plant Biotechnology
Authors : Ara Kirakosyan, Peter B. Kaufman
DOI : https://doi.org/10.1007/978-1-4419-0194-1
Publisher : Springer New York, NY
eBook Packages : Biomedical and Life Sciences , Biomedical and Life Sciences (R0)
Copyright Information : The Editor(s) (if applicable) and The Author(s), under exclusive license to Springer Science+Business Media, LLC, part of Springer Nature 2009
Hardcover ISBN : 978-1-4419-0193-4 Published: 30 July 2009
Softcover ISBN : 978-1-4899-7916-2 Published: 23 August 2016
eBook ISBN : 978-1-4419-0194-1 Published: 15 August 2009
Edition Number : 1
Number of Pages : XIV, 405
Topics : Plant Genetics and Genomics , Plant Sciences
- Publish with us
Policies and ethics
- Find a journal
- Track your research
Academia.edu no longer supports Internet Explorer.
To browse Academia.edu and the wider internet faster and more securely, please take a few seconds to upgrade your browser .
- We're Hiring!
- Help Center
Plant biotechnology
- Most Cited Papers
- Most Downloaded Papers
- Newest Papers
- Last »
- Biotechnology Follow Following
- Plant Molecular Biology Follow Following
- Plant breeding and genetics Follow Following
- Molecular Biology Follow Following
- Plant Tissue Culture Follow Following
- Genetics Follow Following
- Plant Tissue Culture and Genetic Transformation Follow Following
- Plant Breeding Follow Following
- Plant Biotechnology and Molecular Biology Follow Following
- Bioinformatics Follow Following
Enter the email address you signed up with and we'll email you a reset link.
- Academia.edu Journals
- We're Hiring!
- Help Center
- Find new research papers in:
- Health Sciences
- Earth Sciences
- Cognitive Science
- Mathematics
- Computer Science
- Academia ©2024
Thank you for visiting nature.com. You are using a browser version with limited support for CSS. To obtain the best experience, we recommend you use a more up to date browser (or turn off compatibility mode in Internet Explorer). In the meantime, to ensure continued support, we are displaying the site without styles and JavaScript.
- View all journals
Biotechnology articles from across Nature Portfolio
Biotechnology is a broad discipline in which biological processes, organisms, cells or cellular components are exploited to develop new technologies. New tools and products developed by biotechnologists are useful in research, agriculture, industry and the clinic.
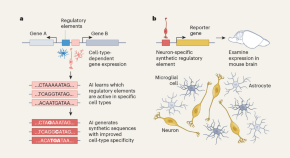
AI-designed DNA sequences regulate cell-type-specific gene expression
Researchers have used artificial-intelligence models to create regulatory DNA sequences that drive gene expression in specific cell types. Such synthetic sequences could be used to target gene therapies to particular cell populations.
- Andreas R. Pfenning

Immunogenic amines on lipid nanoparticles
Amine headgroups in the ionizable lipids of lipid nanoparticles contribute to their immunogenicity.
- Preeti Sharma
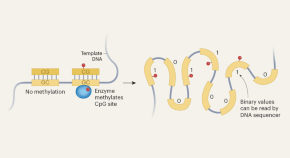
‘Do-it-yourself’ data storage on DNA paves way to simple archiving system
Data can be stored on DNA, but the methods involve time-consuming DNA synthesis and must be done by experts. A user-friendly approach has been developed that potentially solves these problems.
- Carina Imburgia
- Jeff Nivala
Related Subjects
- Animal biotechnology
- Applied immunology
- Assay systems
- Biomaterials
- Biomimetics
- Cell delivery
- Environmental biotechnology
- Expression systems
- Functional genomics
- Gene delivery
- Gene therapy
- Industrial microbiology
- Metabolic engineering
- Metabolomics
- Molecular engineering
- Nanobiotechnology
- Nucleic-acid therapeutics
- Oligo delivery
- Peptide delivery
- Plant biotechnology
- Protein delivery
- Regenerative medicine
- Stem-cell biotechnology
- Tissue engineering
Latest Research and Reviews
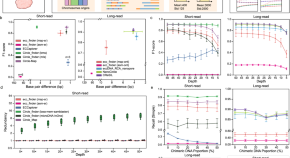
Comparative analysis of methodologies for detecting extrachromosomal circular DNA
Sequencing-based studies have advanced our understanding of the diverse functions of extrachromosomal circular DNA (eccDNA). Here the authors systematically compare the performance of several bioinformatic pipelines and experimental methods that have been developed for eccDNA detection.
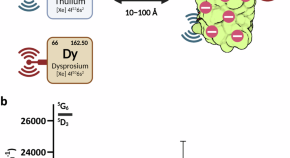
Supercharged fluorescent proteins detect lanthanides via direct antennae signaling
Lanthanides are in high global demand and a sustainable extraction practice is needed to keep pace. Here, Huang and colleagues reengineer fluorescent protein surface charges to bind and detect lanthanides.
- Kevin Y. Huang
- Lizette Cardenas
- David J. F. Walker
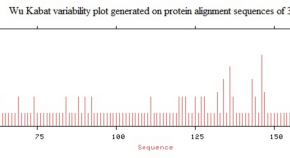
A comprehensive and single-use foot-and-mouth disease sero-surveillance prototype employing rationally designed multiple viral antigens
- Anamica Hossain
- K. M. Mazharul Alam
- Munawar Sultana
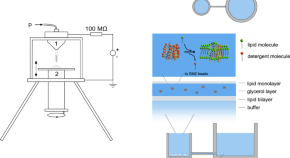
Nanoelectrospray based synthesis of large, transportable membranes with integrated membrane proteins
- Matthias Wilm
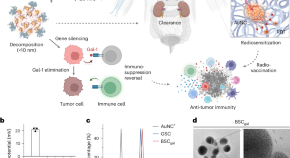
Gold-siRNA supraclusters enhance the anti-tumor immune response of stereotactic ablative radiotherapy at primary and metastatic tumors
Gold-siRNA clusters boost the immune response of radiotherapy against primary and distant tumors.
- Yuyan Jiang
- Hongbin Cao
- Quynh-Thu Le
Long-term lineage commitment in hematopoietic stem cell gene therapy
- Andrea Calabria
- Giulio Spinozzi
- Eugenio Montini
News and Comment
Forming folate-fortified rice.
- Francesco Zamberlan
High-resolution measurement of individual telomere lengths with Telo-seq
In this Tools of the Trade article, Carly Tyer describes the development of Telo-seq, a method to enrich and sequence all telomeres within a sample, and highlights its use in distinguishing between the two telomere maintenance mechanisms used in cancer cells.
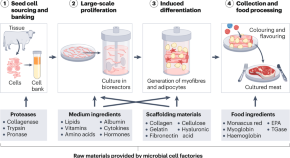
Microbial cell factories for cost-effective and high-quality cultured meat
Commercializing cultured meat requires cost reduction and quality improvement. Microbial cell factories can produce cost-effective and high-quality raw materials for cultured meat production.
- Jingwen Zhou
Quick links
- Explore articles by subject
- Guide to authors
- Editorial policies

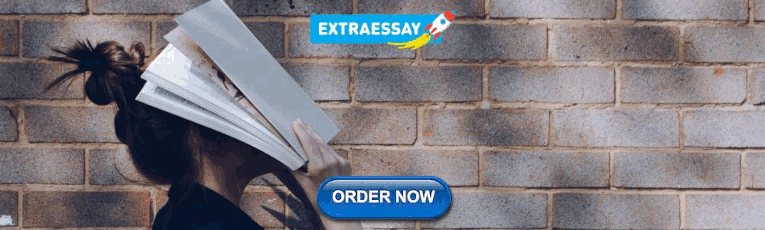
IMAGES
VIDEO
COMMENTS
Plant biotechnology can be defined as the introduction of desirable traits into plants through genetic modification. ... Research Open Access 09 Oct 2024 Nature Communications. Volume: 15, P: 8568.
Read the latest Research articles in Plant biotechnology from Nature Biotechnology. ... Plant biotechnology articles within Nature Biotechnology. Featured. Patents | 14 October 2024.
The Plant Biotechnology section at Frontiers in Plant Science mainly publishes applied studies examining how plants can be improved using modern genetic techniques (Lloyd and Kossmann, 2021). This Research Topic was designed to allow editors from the section to highlight some of their own plant biotechnological work.
Resistance-gene-directed discovery of a natural-product herbicide with a new mode of action. Fungal genome mining targeted to self-resistance genes close to biosynthetic gene clusters identifies a ...
Molecular and Physiological Mechanisms Driving Phytoremediation. Azam Noori. Hamidreza Sharifan. Andrés Rodríguez-Seijo. 1,999 views. 1 article. This section explores all branches of plant biotechnology, addressing the attempts of modern technologies to satisfy increasing demands for crop production.
Explore the latest full-text research PDFs, articles, conference papers, preprints and more on PLANT BIOTECHNOLOGY. Find methods information, sources, references or conduct a literature review on ...
Plant Biotechnology Journal presents research at the forefront of applied plant science and molecular plant sciences. Published in partnership with the Society for Experimental Biology (SEB) and the Association of Applied Biology (AAB) it is dedicated to showcasing original research and insightful reviews by renowned researchers in the field of plant biotechnology.
These aspects have been addressed in the 17 papers published in this Special Issue titled 'Plant Biotechnology and Crop Improvement'. There have been four general review papers covering different biotechnologies and thirteen original research contributions focusing on different crop groups, including tropical and temperate cereal, legume ...
Marker-assisted selection for scab resistance and columnar growth habit in inter-varietal population of apple (Malus × domestica) Plant Biotechnology Reports is a peer-reviewed journal emphasizing fundamental and applied research in plant biotechnology.
Plant Biotechnology Reports is a peer-reviewed journal emphasizing fundamental and applied research in plant biotechnology. Offers comprehensive coverage extending to molecular biology, genetics, biochemistry, and more. Prioritizes studies on plants indigenous to the Asia-Pacific region. Encourages studies related to commercialization of plant ...
order to help the reader to grasp and understand the inherent complexity of plant biotechnology better. The topics covered in this book will be of interest to plant biologists, biochemists, molecular biologists, pharmacologists, and pharmacists; agronomists, plant breed- ... 50 peer-reviewed research papers in professional journals and several ...
endangered world. Marc Van Montagu 1. 1 VIB-International Plant Biotechnology Outreach, Ghent University, Ghent, Belgium. Abstract. This paper draws on the importance of science-based agriculture ...
The Plant Biotechnology section at Frontiers in Plant Science mainly publishes applied studies examining how plants can be improved using modern genetic techniques (Lloyd and Kossmann, 2021). This Research Topic was designed to allow editors from the section to highlight some of their own plant biotechnological work.
Abstract. Biotechnology explores the metabolic properties of living organisms for the production of valuable products of a very different structural and organizational level. Plant serves as an important source of primary and secondary metabolites used in pharmacy, biotechnology, and food technology. Plant biotechnology has gained importance in ...
Background. Over the past decades, the term 'bioinformatics' has become a buzzword in all areas of research in biological science. With the continuous development and advancement in molecular biology, the explosive growth of biological information required a more organized, computerized system to collect, store, manage, and analyse the vast amount of biological data generated in the ...
Abstract. Agriculture is experiencing a technological inflection point in its history, while also facing unprecedented challenges posed by human population growth and global climate changes. Key ...
Dr. Kirakosyan is principal author of over 50 peer-reviewed research papers in professional journals and several chapters in books dealing with plant biotechnology and molecular biology. He is second author of best-selling book, "Natural Products from Plants", 2 nd edition (2006). Ara Kirakosyan is a full member of the Phytochemical Society of ...
A study on pearl millet (Pennisetum glaucum L.) plant Biochemical and histochemical changes inoculated with indigenous AM fungi under Barren soil. The soil organisms that develop beneficial Symbiotic relationships with plants roots and contribute to plant growth are mycorrhizal (AM) fungi.
High levels of superoxide (O 2 • -) are known to regulate plant stem cell behavior, but its downstream effectors remain unclear. O 2 • - was found to directly promote DNA demethylase ROS1 ...
Biotechnology is a broad discipline in which biological processes, organisms, cells or cellular components are exploited to develop new technologies. New tools and products developed by ...