- Open access
- Published: 04 January 2021
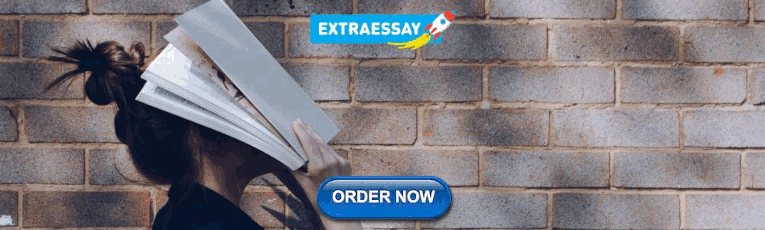
HPTLC analysis of Fumaria parviflora (Lam.) methanolic extract of whole plant
- Anjali Bhargava 1 ,
- Pragya Shrivastava 1 &
- Anita Tilwari 2
Future Journal of Pharmaceutical Sciences volume 7 , Article number: 1 ( 2021 ) Cite this article
7316 Accesses
124 Citations
Metrics details
Fumaria parviflora (Lam.), commonly known as “fine-leaved fumitory,” is well known for its therapeutic properties in the Indian traditional medicinal system. The presence of important bioactive compounds in plants makes them pharmacologically valuable. Therefore, in the present study, the high-performance thin layer chromatography (HPTLC) analysis of Fumaria parviflora (whole plant) methanolic extract was performed for its phytochemical profiling.
The HPTLC densitometric analysis of the methanolic extract of Fumaria parviflora (whole plant) was carried out using CAMAG HPTLC system, and the results were obtained in the form of chromatograms (scanned at the wavelength of 254 nm and 366 nm) representing several peaks. The phytochemical profile of the plant was determined and presented in the tables showing the total number of peaks, peak heights, peak area, percent area, and Rf values.
The study concluded that F. parviflora methanolic extract of the whole plant contains a rich variety of phytochemicals which might be accountable for its therapeutic value and thus justifies its traditional use in India.
Medicinal plants, due to the presence of bioactive phytochemicals, play a very important role in human life for maintaining good health. The use of medicinal herbs in the treatment of infection is an age-old practice, and several natural products are used as phytotherapic for the treatment of many diseases [ 1 ]. The search for a newer source of antibiotics is a global challenge, since many infectious agents are becoming resistant to synthetic drugs [ 2 ]. There are thousands of medicinal plants known to have a long history of usage for their curative properties against various diseases and ailments [ 3 ]. The use of herbal drugs is once more escalating in the form of Complementary and Alternative Medicine (CAM) [ 4 ].
Fumaria parviflora Lam., commonly known as fine-leaved fumitory (in English), Shahatra, Pittapapara, or Pittapapada (in Hindi), belongs to the family Fumariaceae. Fumaria parviflora (Fumariaceae) is a pale green, diffuse, much branched annual herb widely used in Ayurvedic medicine as well as in traditional Yunani system of medicine throughout India [ 5 ]. The entire herb is traditionally used in leprosy, fever [ 6 ], and detoxification and as laxative, diuretic, and diaphoretic [ 7 ].
The World Health Organization (WHO) has stressed on the need for scientific validity of herbal drugs and ensuring, devising, and implementing sound science [ 8 ]. Several techniques are available for the qualitative and quantitative estimation of phytochemicals present in plants. Nowadays, new technology has made it possible to identify, screen, and isolate these active compounds [ 9 ]. The HPTLC (high-performance thin layer chromatography) is an advanced form of TLC as it provides high resolution and much accurate data. It is accepted all over the world as one of the most powerful analytical techniques used for phytochemical and biomedical analysis. It is an inexpensive, simple, and rapid method for the estimation of chemical components present in test sample and therefore most widely used by pharmaceutical industries for new drug discovery. The present study was performed for the phytochemical profiling of Fumaria parviflora (whole plant) methanolic extract by the HPTLC technique.
The plant material was washed and then kept for shade drying for 7 days. The dried plant sample was powdered by mechanical grinder into a fine powder. The air-dried powdered material of the whole plant of Fumaria parviflora (100 g) was extracted with hydroalcoholic solvent [methanol and water solvent (1:1 v/v)] using the Soxhletion process with the help of a Soxhlet apparatus. Excess solvent was then evaporated in a water bath at 50–100 °C to obtain the crude and stored in airtight containers.
Instrumentation
A CAMAG HPTLC system equipped with LINOMAT 5 applicator fitted with 100 μl syringe, CAMAG TLC scanner, and winCATS software was used.
Chemicals and solvents
All the solvents used were of chromatography grade, and all the chemicals used were of analytical reagent grade.
Preparation of samples
Dried extract (10 g) of F. parviflora was dissolved in 100 ml HPTLC grade methanol and filtered. This solution was used as a test solution for the HPTLC study.
Chromatographic conditions
The HPTLC was performed on 7.0 × 10.0 cm precoated silica gel 60 F 254 HPTLC plate (E. MERCK KGaA). No pre-washing and modification of the plate were done. The sample solution was applied as bands to the plate by CAMAG Linomat applicator fitted with 100 μl syringe (Table 1 ). The stable application rate was 150 nl/s. The sample loaded plate was kept in automatic development chamber with mobile phase—chloroform:ethyl acetate:formic acid (5:4:1 v/v/v). Densitometric scanning was performed with CAMAG TLC scanner-4 equipped with winCATS software. The bands were visualized using CAMAG visualizer, and the images were captured in white light and 254 nm (short UV) and 366 nm (long UV) wavelengths (Table 2 ). When exposed to short-wave UV light of 254 nm, UV-active compounds will undergo fluorescence quenching and appear as dark spots on a bright background. Conversely, compounds that absorb 366 nm UV light will appear as bright spots on a dark background [ 10 ].
The HPTLC analysis of F. parviflora Lam. revealed the presence of various phytochemicals as illustrated in the figures and tables below. The chromatograms (Figs. 1 , 2 , 3 , and 4 ) were obtained upon scanning at UV 254 nm and 366 nm, and peak tables were generated. The Rf values, peak height, peak area, and percent area of the unknown substances are depicted in the tables (Tables 3 , 4 , 5 , and 6 ).
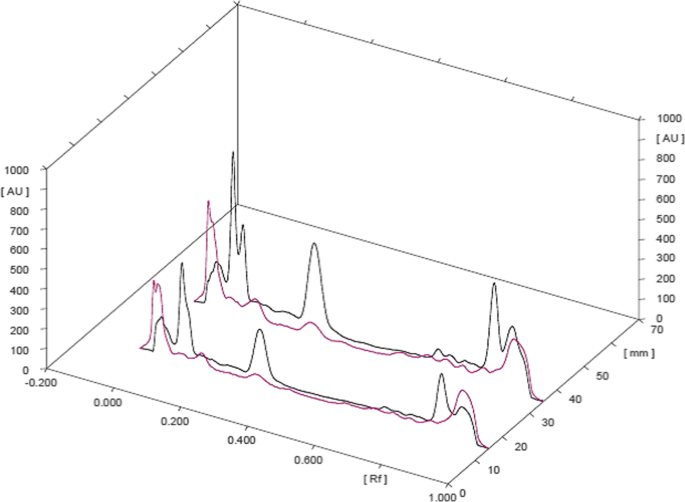
3D overlay of HPTLC chromatogram of all tracks, at all wavelengths
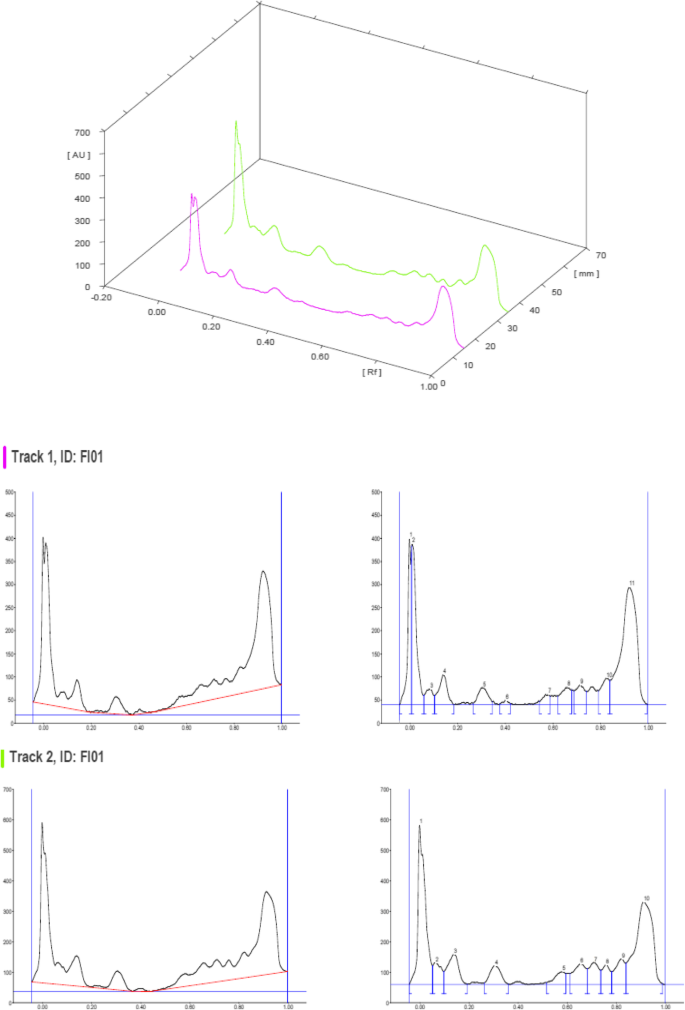
HPTLC chromatograms scanned at 254 nm
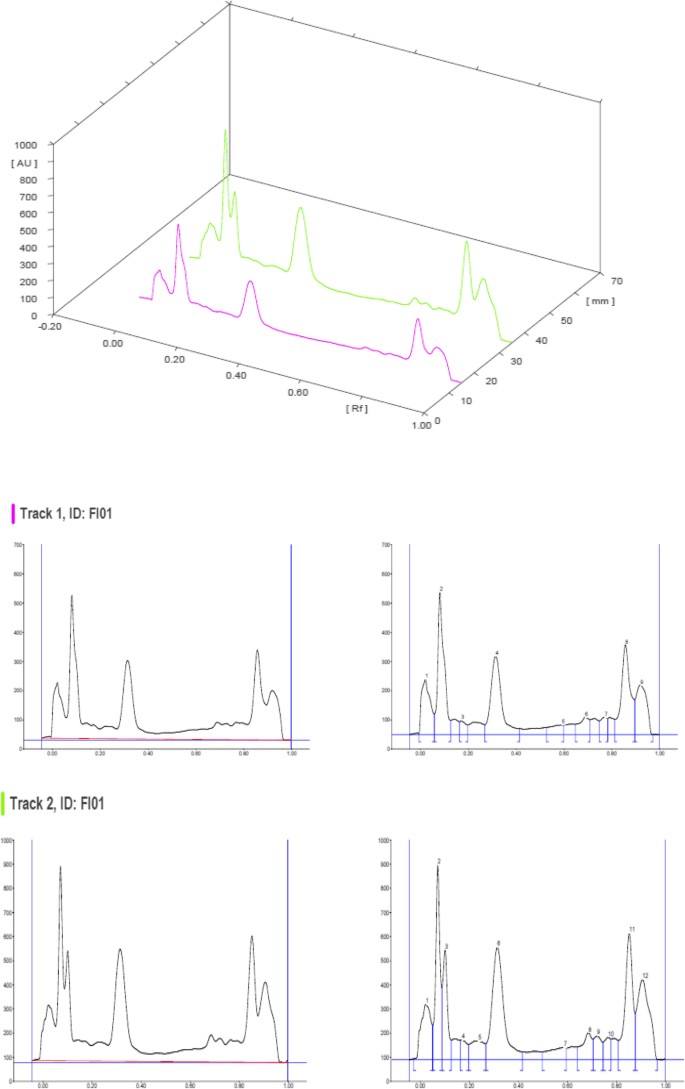
HPTLC chromatograms scanned at 366 nm
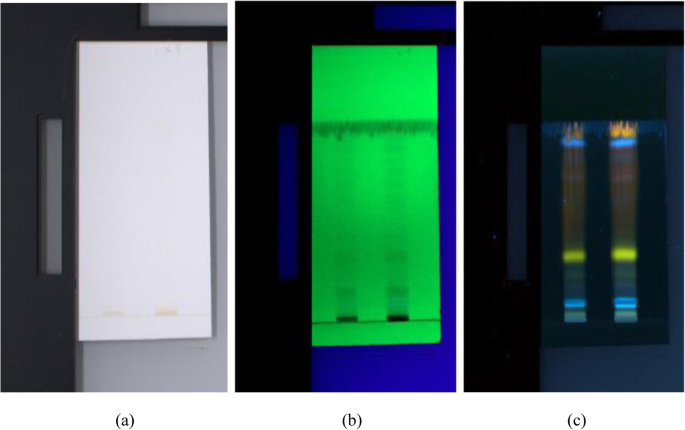
HPTLC chromatograms visualized under a white light, b UV 254 nm, and c UV 366 nm
The HPTLC performed on the methanolic extract of Fumaria parviflora (Lam.) showed the presence of various phytoconstituents in different concentrations as illustrated in figures and tables. Figure 1 represents the 3-dimensional overlay of the chromatogram of all tracks, at all measured wavelengths. The chromatogram scanned at 254 nm (Fig. 2 ) represents 11 and 10 peaks for track 1 and track 2, respectively, whereas the chromatogram scanned at 366 nm (Fig. 3 ) indicates 9 and 12 peaks for track 1 and track 2, respectively. The number of peaks indicates the presence of different phytoconstituents present in the sample. The Rf values (Tables 3 , 4 , 5 , and 6 ) calculated for the phytoconstituents present in the tested sample would be helpful in the identification of the unknown compounds by comparing them with the reference standards, and from the values of peak area, the concentration of the compounds can be determined. The bands of separated compounds can be seen (Fig. 4 ) on the TLC plates visualized under white light and UV of wavelengths 254 nm and 366 nm.
It has been reported from the previous studies that a wide range of bioactive compounds of medicinal significance are present in various species of Fumaria . The HPTLC study conducted on Fumaria vaillantii showed the presence of protopine and rutin in methanol extract of the whole plant at Rf 0.51 and 0.26, respectively [ 11 ]. Some of the Fumaria species are known to exhibit antifungal [ 12 ], antibacterial [ 13 ], and anti-inflammatory [ 14 ] activities due to the presence of bioactive phytochemicals such as alkaloids, polyphenols, and flavonoids. Thus, from the earlier researches, it is evident that various species of Fumaria contain some bioactive compounds important for pharmaceutical industries.
The findings of the present study are limited to the HPTLC analysis of Fumaria parviflora methanolic extract to estimate the presence of different phytochemicals from the chromatogram peaks and obtain the peak tables; however, the identification of the unknown phytochemicals is not done.
The present study revealed the presence of several phytochemicals in F. parviflora which might be the cause for its healing properties and thus justifies its usage as a remedy in various ailments. New drug formulations require the isolation and identification of important phyto-compounds possessing pharmacological properties. The HPTLC study carried out for F. parviflora chemical profiling will be helpful in the identification of bioactive compounds and markers, by comparing the Rf values of the compounds with the reference standards.
Availability of data and materials
All data and material are available upon request.
Abbreviations
Retention factor
High-performance thin layer chromatography
Ultraviolet
Hydrargyrum (mercury)
Sisodiya D, Shrivastava P (2018) Antimicrobial activity of Euphorbia thymifolia (L.) and Manilkara hexandra (Roxb.). Int J Curr Adv Res 7(2):9660–9663
Google Scholar
Latha SP, Kannabiran K (2006) Antimicrobial activity and phytochemicals of Solanum trilobatum Linn. Afr J Biotechnol 5:2402–2404
Sisodiya D, Shrivastava P (2018) Phytochemical screening, thin layer chromatography and quantitative estimation of bioactive constituents in aqueous extract of Manilkara hexandra (Roxb.) dubard. Int J Recent Sci Res 9(1):23083–23086
Cooper LN, Blais BS (2004) Theory of cortical plasticity. World scientific publishing, Singapore
Book Google Scholar
Kumar S, Sharma AK, Kamboj A (2017) Fumaria parviflora Lam. (Fumitory): a traditional herbal medicine with modern evidence. Asian J Pharm Pharmacol 3(6):200–207
CAS Google Scholar
Anonymous (2004) The ayurvedic pharmacopoeia of India, 1 Ministry of Health and Family Welfare, New Delhi, Government of India, 84–86.
Anonymous (2007) Fumaria parviflora Lam. In: Khare CP (ed) Indian medicinal plants. Springer, Heidelberg, p 275
Tilburt JC, Kaptchuk TJ (2008) Herbal medicine research and global health: an ethical analysis. Bull World Health Organ 86:594–599
Article Google Scholar
Sisodiya D, Shrivastava P (2017) Qualitative and quantitative estimation of bioactive compounds of Euphorbia thymifolia L. Asian J Pharm Edu Res 6(3):34–43
Thin-layer chromatography evaluation. https://www.merckmillipore.com . Accessed 6 Nov 2020.
Upadhye AS, Rajopadhye AA (2011) Botanical and phytochemical standardization of Fumaria vaillantii Loisel. Indian J Nat Prod Resour 2(3):369–374
Moghtader M (2013) In vitro antifungal effects of Fumaria vaillantii Loisel. essential oil on Aspergillus flavus . J Yeast Fungal Res 4:21–25
Khamtache-Abderrahima S, Lequart-Pillonb M, Gontierb E, Gaillardb I, Pilardb S et al (2016) Isoquinoline alkaloid fractions of Fumaria officinalis : characterization and evaluation of their antioxidant and antibacterial activities. Ind Crops Prod 94:1001–1008
Bribi N, Algieri F, Rodriguez-Nogales A, Vezza T, Garrido-Mesa J et al (2016) Intestinal anti-inflammatory effects of total alkaloid extract from Fumaria capreolata in the DNBS model of mice colitis and intestinal epithelial CMT93 cells. Phytomedicine 23:901–913
Article CAS Google Scholar
Download references
Acknowledgements
The authors are thankful to Vindhya Herbal Testing & Research Laboratory, Bhopal, Madhya Pradesh, for providing technical facilities and assistance required for this work.
Collection, identification, and authentication of plant
The plant material was collected in the month of July 2020 and identified taxonomically by Dr. Suman Mishra, Consultant Taxonomist, Xcellventure Institute of Fundamental Research Pvt. Ltd., Bhopal (MP). She is also a botany scientist in MFP-PARC, Barkheda Pathani, Bhopal. The plant was identified and authenticated as Fumaria parviflora Lam. belonging to the family Fumariaceae by its macroscopic, microscopic, and powder microscopic examination.
No funding was received for this research.
Author information
Authors and affiliations.
Department of Life Science, Rabindranath Tagore University, Village-Mendua, Post-Bhojpur, Distt. Raisen, Bhopal, Madhya Pradesh, 464993, India
Anjali Bhargava & Pragya Shrivastava
Madhya Pradesh Council of Science and Technology, Bhopal, Madhya Pradesh, India
Anita Tilwari
You can also search for this author in PubMed Google Scholar
Contributions
AB executed the work and prepared the manuscript. PS planned the work and provided proper guidance for the research. AT contributed to the research design and edited the manuscript. All the authors have read and approved the manuscript.
Corresponding author
Correspondence to Anjali Bhargava .
Ethics declarations
Ethics approval and consent to participate.
Not applicable.
Consent for publication
Competing interests.
The authors declare that they have no competing interests.
Additional information
Publisher’s note.
Springer Nature remains neutral with regard to jurisdictional claims in published maps and institutional affiliations.
Rights and permissions
Open Access This article is licensed under a Creative Commons Attribution 4.0 International License, which permits use, sharing, adaptation, distribution and reproduction in any medium or format, as long as you give appropriate credit to the original author(s) and the source, provide a link to the Creative Commons licence, and indicate if changes were made. The images or other third party material in this article are included in the article's Creative Commons licence, unless indicated otherwise in a credit line to the material. If material is not included in the article's Creative Commons licence and your intended use is not permitted by statutory regulation or exceeds the permitted use, you will need to obtain permission directly from the copyright holder. To view a copy of this licence, visit http://creativecommons.org/licenses/by/4.0/ .
Reprints and permissions
About this article
Cite this article.
Bhargava, A., Shrivastava, P. & Tilwari, A. HPTLC analysis of Fumaria parviflora (Lam.) methanolic extract of whole plant. Futur J Pharm Sci 7 , 1 (2021). https://doi.org/10.1186/s43094-020-00150-x
Download citation
Received : 23 September 2020
Accepted : 30 November 2020
Published : 04 January 2021
DOI : https://doi.org/10.1186/s43094-020-00150-x
Share this article
Anyone you share the following link with will be able to read this content:
Sorry, a shareable link is not currently available for this article.
Provided by the Springer Nature SharedIt content-sharing initiative
- Fumaria parviflora
- Methanolic extract
- Densitometry
- Chromatogram

Paper Chromatography of Plant Pigments
Learning Objectives
After completing the lab, the student will be able to:
- Extract pigments from plant material.
- Separate pigments by paper chromatography.
- Measure R f (retention factor) values for pigments.
Activity 2: Pre-Assessment
- The leaves of some plants change color in fall. Green foliage appears to turn to hues of yellow and brown. Does the yellow color appear because carotenoids replace the green chlorophylls? Explain your reasoning.
- Examine the molecular structures of photosynthetic pigments in Figure 10.1. Photosynthetic pigments are hydrophobic molecules located in thylakoid membranes. Will these pigments dissolve in water?
Activity 2: Paper Chromatography of Plant Pigments
Paper chromatography is an analytical method that separates compounds based on their solubility in a solvent.
The solvent is used to separate a mixture of molecules that have been applied to filter paper. The paper, made of cellulose, represents the stationary or immobile phase. The separation mixture moves up the paper by capillary action. It is called the mobile phase. The results of chromatography are recorded in a chromatogram. Here, the chromatogram is the piece of filter paper with the separated pigment that you will examine at the end of your experiment (see Figure 10.4).
We separate the compounds based on how quickly they move across the paper. Compounds that are soluble in the solvent mixture will be more concentrated in the mobile phase and move faster up the paper. Polar compounds will bind to the cellulose in the paper and trail behind the solvent front. As a result, the different compounds will separate according to their solubility in the mixture of organic solvents we use for chromatography.
This video demonstrates the principles and examples of chromatography. You will experiment with only paper chromatography in this lab; however, you will see that you are already familiar with some uses of thin layer chromatography.
Safety Precautions
- Work under a hood or in a well-ventilated space and avoid breathing solvents.
- Do not have any open flames when working with flammable solvents.
- Wear aprons and eye protection.
- Do not pour any organic solvent down the drain.
- Dispose of solvents per local regulations.
- Use forceps to handle chromatography paper that has been immersed in solvent and wash your hands after completing this activity.
For this activity, you will need the following:
- Plant material: intact leaves of spinach and Coleus (one leaf of each plant per pair of students)
- Filter or chromatography paper
- Ruler (one per group)
- Colored pencils
- Beakers (400 mL) (Mason jars are an acceptable substitute)
- Aluminum foil
- Petroleum ether: acetone: water in a 3:1:1 proportion
- If no hood or well-ventilated place is available, the mixture can be substituted with 95 percent isopropyl alcohol. Note that, if isopropyl alcohol is used, the pigment bands will smear. You may not be able to separate and identify the chlorophylls or carotene from xanthophyll.
For this activity, you will work in pairs .
Structured Inquiry
Step 1: Hypothesize/Predict: Discuss with your lab partner what color pigments will likely be present in the spinach leaves. Write your predictions in your lab notebook and draw a diagram of how you think the pigments will separate out on the chromatography paper.
Step 2: Student-led Planning: Read step 3 below. Discuss with your lab partner the setup of the experiment. Then agree upon the dimensions of the filter/chromatography paper that you will use. To allow good separation, the paper should not touch the walls of the container. The paper must fit inside the container while being long enough for maximum separation. Write all your calculations in your lab notebook.
Step 3: Follow the steps below to set up your filter paper and perform the chromatography experiment.
- Prepare the chromatogram by cutting a piece of filter paper. Transfer pigments from spinach leaves as in Activity 1. A heavy application line will yield stronger colors when the pigments separate, making it easier to read results. Allow the pigments to dry between applications. Wet extracts diffuse on the paper and yield blurry lines.
- Form a cylinder with the filter paper without overlapping the edges (to avoid edge effects). The sample should face the outside of the cylinder. Secure the top and bottom of the cylinder with staples.
- Pour enough separation mixture to provide a mobile phase while staying below the origin line on the chromatogram. The exact volume is not critical if the origin, the start line where you applied the solvent, is above the solvent. See Figure 10.4.
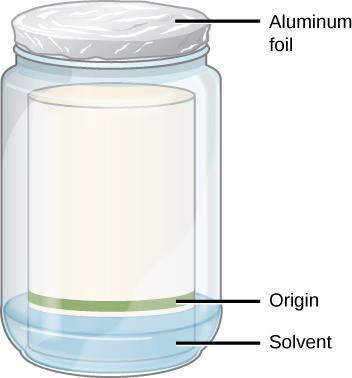
- Label the beaker with a piece of tape with your initials and your partner’s initials.
- Lower the paper into the container with the band from the extraction in the lower section. The paper must touch the solvent, but not reach the band of pigment you applied. Why must the band be above the solvent line? Write your answer in your notebook.
- Cover the container tightly with a piece of aluminum foil.
- Track the rising of the solvent front. Can you see a separation of colors on the paper?
- When the solvent front is within 1 cm of the upper edge of the paper, remove the cylinder from the beaker using forceps. Trace the solvent front with a pencil before it evaporates and disappears! Draw the colored bands seen on your chromatography paper in your lab notebook immediately. The colors will fade upon drying. If no colored pencils are available, record the colors of the lines.
- Let the paper dry in a well-ventilated area before making measurements because the wet paper is fragile and may break when handled. This is also a precaution to avoid breathing fumes from the chromatogram.
- Discard solvent mixture per your instructor’s directions. Do not pour down the drain.
Step 4: Critical Analysis: Open the dried cylinder by removing the staples. Measure the distance from the first pencil line to the solvent front, as shown in Figure 10.5. This is the distance traveled by the solvent front. Measure the distance from the pencil line to the middle point of each color band and the original pencil line. Record your results in your notebook in a table modeled after Table 10.1. The retention factor (R f ) is the ratio of the distance traveled by a colored band to the distance traveled by the solvent front. Calculate R f values for each pigment using the following equation:
R f=Distance traveled by colored band/Distance traveled by solvent front
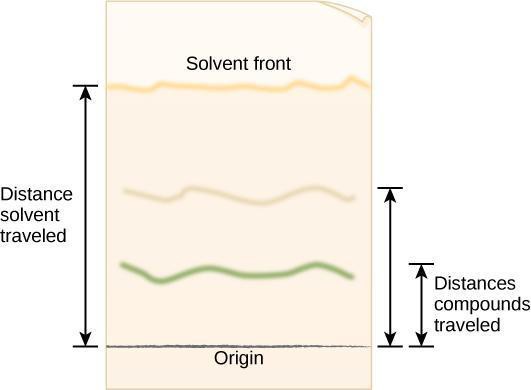
Step 5: After determining the color of the band, tentatively identify each band. Did your results support your hypothesis about the color of each band? Discuss which aspects of the experiments may have yielded inconclusive results. How could you improve the experiment?
Guided Inquiry
Step 1: Hypothesize/Predict: What type of pigments are present in Coleus leaves and where are the different colors located? Can you make a hypothesis based on the coloration of the variegated leaves? Write your hypothesis down in your lab notebook. Would there be a difference if you performed chromatography on pigment composition from different colored regions of the leaves?
Step 2: Student-led Planning: Cut the chromatography/filter paper to the dimensions needed. Apply pigments from different parts of the Coleus leaves following the procedure described under Activity 1, keeping in mind that a darker line will yield stronger colors when the pigments are separated, which will make it easier to read the results. Allow the pigments to dry between applications. Wet extracts diffuse on the paper and yield blurry lines.
Step 3: When the solvent front reaches 1 cm from the top of the filter paper, stop the procedure. Draw the pigment bands you see on the filter paper in your lab notebook. Clearly indicate the color you observed for each band.
Step 4: Let the cylinder dry and measure the distance the front traveled from the origin and the distances traveled by each of the pigments. If the bands broadened during separation, take measurements to the middle of each band.
Step 5: Critical Analysis: Calculate R f for each of the bands and record them in a table in your notebook. Compare the R f you obtained with those of other groups. Are the R f values similar? What may have altered R f values?
Assessments
- Carotenoids and chlorophylls are hydrophobic molecules that dissolve in organic solvents. Where would you find these molecules in the cell? What would happen if you ran the chromatography in this lab with water as the solvent?
- All chlorophyll molecules contain a complexed magnesium ion. Your houseplant is developing yellow leaves. What may cause this, and how can you restore your plant’s health?
- Seeds that grow under dim light are said to be etiolated, which describes their pale and spindly appearance. They soon waste away after exhausting their food reserves. Can you explain this observation?
Lab Manual for Biology Part I Copyright © 2022 by LOUIS: The Louisiana Library Network is licensed under a Creative Commons Attribution-NonCommercial-ShareAlike 4.0 International License , except where otherwise noted.
Share This Book
- Search Menu
- Advance articles
- Author Guidelines
- Submission Site
- Open Access Policy
- Self-Archiving Policy
- About Journal of AOAC INTERNATIONAL
- About AOAC INTERNATIONAL
- Editorial Board
- Advertising & Corporate Services
- Journals on Oxford Academic
- Books on Oxford Academic
Article Contents
- < Previous
The Role of Planar Chromatography in Medicinal Plant Research
- Article contents
- Figures & tables
- Supplementary Data
Szabolcs Nyiredy, The Role of Planar Chromatography in Medicinal Plant Research, Journal of AOAC INTERNATIONAL , Volume 84, Issue 4, 1 July 2001, Pages 1219–1231, https://doi.org/10.1093/jaoac/84.4.1219
- Permissions Icon Permissions
This paper summarizes the role of planar chromatography (PC) in medicinal and aromatic plant (MAP) research and development, and demonstrates the importance of the technique, after extraction, in the analysis of MAP (identification and quantitative determination of the separated compound/s), in the purification and isolation process, and in different types of screening procedure. Special attention is paid to analytical, micropreparative and preparative forced-flow techniques, for example overpressured-layer chromatography (OPLC) and rotation planar chromatography (RPC). The special features of analytical, micropreparative, and preparative layer chromatography (PLC), OPLC, and RPC are compared in tables. Purification and isolation procedures using forced-flow techniques are shown in flowcharts. Some applications, relating to different classes of substance, are presented to demonstrate the versatility of various planar chromatographic techniques.
Email alerts
Citing articles via.
- Recommend to Your Librarian
- Advertising and Corporate Services
- Journals Career Network
Affiliations
- Online ISSN 1944-7922
- Print ISSN 1060-3271
- Copyright © 2024 AOAC INTERNATIONAL
- About Oxford Academic
- Publish journals with us
- University press partners
- What we publish
- New features
- Open access
- Institutional account management
- Rights and permissions
- Get help with access
- Accessibility
- Advertising
- Media enquiries
- Oxford University Press
- Oxford Languages
- University of Oxford
Oxford University Press is a department of the University of Oxford. It furthers the University's objective of excellence in research, scholarship, and education by publishing worldwide
- Copyright © 2024 Oxford University Press
- Cookie settings
- Cookie policy
- Privacy policy
- Legal notice
This Feature Is Available To Subscribers Only
Sign In or Create an Account
This PDF is available to Subscribers Only
For full access to this pdf, sign in to an existing account, or purchase an annual subscription.
Thank you for visiting nature.com. You are using a browser version with limited support for CSS. To obtain the best experience, we recommend you use a more up to date browser (or turn off compatibility mode in Internet Explorer). In the meantime, to ensure continued support, we are displaying the site without styles and JavaScript.
- View all journals
- Explore content
- About the journal
- Publish with us
- Sign up for alerts
- Perspective
- Published: 08 July 2021
Mass spectrometry-based metabolomics: a guide for annotation, quantification and best reporting practices
- Saleh Alseekh ORCID: orcid.org/0000-0003-2067-5235 1 , 2 ,
- Asaph Aharoni ORCID: orcid.org/0000-0002-6077-1590 3 ,
- Yariv Brotman 4 ,
- Kévin Contrepois ORCID: orcid.org/0000-0001-9678-5161 5 ,
- John D’Auria ORCID: orcid.org/0000-0002-4865-3938 6 ,
- Jan Ewald ORCID: orcid.org/0000-0002-9415-2317 7 ,
- Jennifer C. Ewald ORCID: orcid.org/0000-0003-1822-4988 8 ,
- Paul D. Fraser 9 ,
- Patrick Giavalisco 10 ,
- Robert D. Hall ORCID: orcid.org/0000-0002-5786-768X 11 , 12 ,
- Matthias Heinemann ORCID: orcid.org/0000-0002-5512-9077 13 ,
- Hannes Link 14 ,
- Jie Luo 15 ,
- Steffen Neumann 16 ,
- Jens Nielsen ORCID: orcid.org/0000-0002-9955-6003 17 , 18 ,
- Leonardo Perez de Souza ORCID: orcid.org/0000-0002-7200-8808 1 ,
- Kazuki Saito ORCID: orcid.org/0000-0001-6310-5342 19 , 20 ,
- Uwe Sauer ORCID: orcid.org/0000-0002-5923-0770 21 ,
- Frank C. Schroeder ORCID: orcid.org/0000-0002-4420-0237 22 ,
- Stefan Schuster 7 ,
- Gary Siuzdak ORCID: orcid.org/0000-0002-4749-0014 23 ,
- Aleksandra Skirycz ORCID: orcid.org/0000-0002-7627-7925 1 , 22 ,
- Lloyd W. Sumner ORCID: orcid.org/0000-0002-4086-663X 24 ,
- Michael P. Snyder ORCID: orcid.org/0000-0003-0784-7987 5 ,
- Huiru Tang ORCID: orcid.org/0000-0002-7139-2756 25 ,
- Takayuki Tohge 26 ,
- Yulan Wang ORCID: orcid.org/0000-0002-2831-8737 27 ,
- Weiwei Wen ORCID: orcid.org/0000-0002-3413-4766 28 ,
- Guowang Xu ORCID: orcid.org/0000-0003-4298-3554 29 ,
- Nicola Zamboni ORCID: orcid.org/0000-0003-1271-1021 21 &
- Alisdair R. Fernie ORCID: orcid.org/0000-0001-9000-335X 1 , 2
Nature Methods volume 18 , pages 747–756 ( 2021 ) Cite this article
69k Accesses
374 Citations
115 Altmetric
Metrics details
Mass spectrometry
- Metabolomics
Mass spectrometry-based metabolomics approaches can enable detection and quantification of many thousands of metabolite features simultaneously. However, compound identification and reliable quantification are greatly complicated owing to the chemical complexity and dynamic range of the metabolome. Simultaneous quantification of many metabolites within complex mixtures can additionally be complicated by ion suppression, fragmentation and the presence of isomers. Here we present guidelines covering sample preparation, replication and randomization, quantification, recovery and recombination, ion suppression and peak misidentification, as a means to enable high-quality reporting of liquid chromatography– and gas chromatography–mass spectrometry-based metabolomics-derived data.
You have full access to this article via your institution.
Similar content being viewed by others
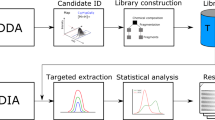
DIAMetAlyzer allows automated false-discovery rate-controlled analysis for data-independent acquisition in metabolomics
Oliver Alka, Premy Shanthamoorthy, … Hannes L. Röst
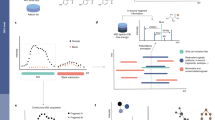
HERMES: a molecular-formula-oriented method to target the metabolome
Roger Giné, Jordi Capellades, … Oscar Yanes
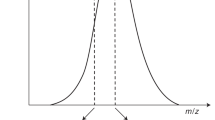
Ultra-high-performance liquid chromatography high-resolution mass spectrometry variants for metabolomics research
Leonardo Perez de Souza, Saleh Alseekh, … Alisdair R. Fernie
Metabolomics, the large-scale study of the metabolic complement of the cell 1 , 2 , 3 , is a mature science that has been practiced for over 20 years 4 . Indeed, it is now a commonly used experimental systems biology tool with demonstrated utility in both fundamental and applied aspects of plant, microbial and mammalian research 5 , 6 , 7 , 8 , 9 , 10 , 11 , 12 , 13 , 14 , 15 . Among the many thousands of studies published in this area over the last 20 years, notable highlights 5 , 6 , 7 , 8 , 10 , 11 , 16 are briefly described in Supplementary Note 1 .
Despite the insight afforded by such studies, the nature of metabolites, particularly their diversity (in both chemical structure and dynamic range of abundance 9 , 12 ), remains a major challenge with regard to the ability to provide adequate coverage of the metabolome that can complement that achieved for the genome, transcriptome and proteome. Despite these comparative limitations, enormous advances have been made with regard to the number of analytes about which accurate quantitative information can be acquired, and a vast number of studies have yielded important biological information and biologically active metabolites across the kingdoms of life 14 . We have previously estimated that upwards of 1 million different metabolites occur across the tree of life, with between 1,000 and 40,000 estimated to occur in a single species 4 .
However, thus far, even the most comprehensive methods cannot provide firm upper limits for metabolite number. Current capabilities for detection and quantification of metabolites fall a long way short of being comprehensive. Currently, combinations of the most comprehensive methods are able to quantify 700 of the 3,700 metabolites predicted to be present in Escherichia coli 17 , 18 , 500 of the 2,680 metabolites predicted to be present in yeast 19 , 20 , 8,000 of the 114,100 metabolites predicted to be present in humans 21 and only 14,000 of the over 400,000 metabolites predicted to be present in the plant kingdom 4 , 22 . Chemical diversity, rapid turnover times and broad dynamic range in cellular abundance currently prohibit the possibility of using single-extraction and single-analysis procedures to measure all metabolites 9 . Consequently, many different extraction techniques and combinations of analytical methods have been developed in an attempt to achieve adequate metabolite coverage. This renders the establishment of good working practices 13 , 15 , 23 , 24 , 25 , 26 more difficult than with RNA-seq 27 , for example. Furthermore, rigorous standards are needed for normalization of metabolomics data 28 , 29 . This is exacerbated by the breadth of aims associated with the measurement of metabolites, which encompass targeted metabolite analysis, metabolite profiling, flux profiling, metabolomics-scale analysis and metabolite fingerprinting techniques 30 , 31 .
Given the myriad of aims and methodologies, we argue that it is particularly important to define clear guidelines for acquisition and reporting of metabolite data because there are many potential sources of misinterpretation. This is not the first time such guidelines have been suggested, with several insightful papers published on this topic 12 , 32 and long-established metabolome databases including MetaboLights 33 , 34 , 35 , 36 and the Metabolome Workbench ( https://www.metabolomicsworkbench.org/ ) also driving this field. A more detailed description of these repositories as well as of more recent developments is provided in Supplementary Note 2 . Although the detailed standards set out by the Metabolomics Standards Initiative 32 and these repositories are laudable and clearly represent the gold standard of metabolomics reporting, it is notable that only a small fraction of published metabolomics studies follow these standards in their entirety and submit their data to the metabolome databases. There are probably several reasons underlying this. First, few journals currently mandate that data be stored in one of the metabolomics repositories. Second, unlike the situation 20 years ago, or even when the work of the Metabolomics Standards Initiative was first published some 13 years ago 32 , 36 , 37 , 38 , metabolomics experiments often represent only one component of studies integrating a wide range of techniques. Moreover, many groups outsource their metabolomics workflow to service providers and do not always have the experience to provide the raw data or even have access to them. In parallel, requiring reviewers to comment on all aspects of multiomics studies in the absence of clear guidelines is a big ask, especially considering that many biologists lack expert competence in the area of metabolomics. Finally, and perhaps most tellingly, there is difficulty in reporting chromatogram-level information, which often requires several attempts to fulfil the criteria of the major metabolomics repositories. However, while the reporting of this information is highly useful for several purposes, it is not essential for all. As we illustrate here, evaluation of the quality of the metabolomics data presented in a paper can effectively be performed on the basis of a relatively small amount of metadata—namely, by analyzing the quality of the metabolite annotation as well as assessing the quantitative recovery of analyte peaks.
Our aim here is to present a simplified reporting workflow, with the hope of capturing more of the missing information. While nuclear magnetic resonance (NMR) and capillary electrophoresis–mass spectrometry (CE–MS) have specific advocates and have clear advantages in structure elucidation and sensitivity, respectively, we will focus here on chromatography (either gas chromatography (GC) or liquid chromatography (LC)) hyphenated to MS; we therefore focus our guidelines on such techniques, given that the majority of metabolomics studies rely on these approaches. In contrast to the suggestions of the Metabolomics Standards Initiative 32 , 36 , 37 , 38 and the major repositories mentioned above, we provide reporting guidelines at the level of the processed data (supported by the provision of representative chromatograms allowing the assessment of metabolite identification), rather than the raw chromatograms. A similar recommendation was made to the plant research community in 2011 (ref. 39 ). Here we have aimed to revise and update these recommendations to (1) be more globally applicable and (2) reinforce our contention that quantification control experiments should be regarded as mandatory and can aid in determining how problematic the effects of ion suppression are in an experiment. We highlight potential sources of error and provide recommendations for ensuring the robustness of the metabolite data obtained and reported. We also present guidelines for sampling, extraction and storage, metabolite identification and reporting. We stress the need for recombination and recovery experiments aimed at checking both qualitative metabolite identifications and the quantitative recovery of these metabolites. In addition, we suggest a stricter nomenclature for metabolite annotation that would improve reporting by removing much of the ambiguity concerning the quality of metabolite annotation that is currently apparent in many metabolomics studies. The scope of our guidelines does not encompass detailed downstream computational analysis of the acquired datasets, although we note several important recent advances in this area 40 , 41 , 42 , 43 , 44 , 45 , 46 , 47 . These tools and their application are discussed in Supplementary Note 3 .
We believe that such efforts are necessary to enable between-laboratory comparisons of datasets, which, as has been demonstrated for transcriptomics, provides huge statistical power and deeper biological insights and, furthermore, provides a basis for better integration with other datasets 48 , 49 .
Sampling, quenching, metabolite extraction and storage
The very first (and particularly vital) step in a metabolomics workflow (Figs. 1 and 2 ) is the rapid stopping, or quenching, of metabolism and extraction of the metabolites in a manner that produces a stable extract that is quantitatively reflective of the endogenous metabolite levels present in the original living cell. This is especially important in highly metabolically active systems such as cells and tissues, but less so in biofluids such as serum, plasma or urine samples 12 . Indeed, there is no one method to fit all cases, with specific sampling, quenching and extraction needed for each tissue type. That said, certain evaluations of quality are universally applicable, and our aim here is to provide clear instructions on how to apply them.
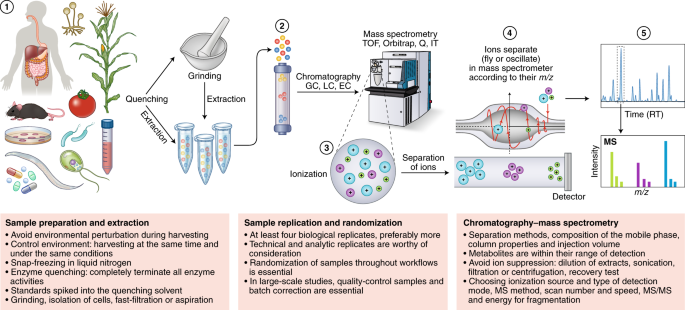
Metabolomics involves several basic steps: (1) sample preparation and extraction; (2) metabolite separation on a column (chromatography) such as by GC, LC or EC; (3) ionization of metabolites using an ion source; (4) separation by a mass analyzer as ions fly or oscillate on the basis of their mass-to-charge ( m / z ) ratio; and (5) detection. Metabolites can be identified on the basis of a combination of retention time (RT) and MS signature. TOF, time of flight; Q, quadrupole; IT, ion trap.
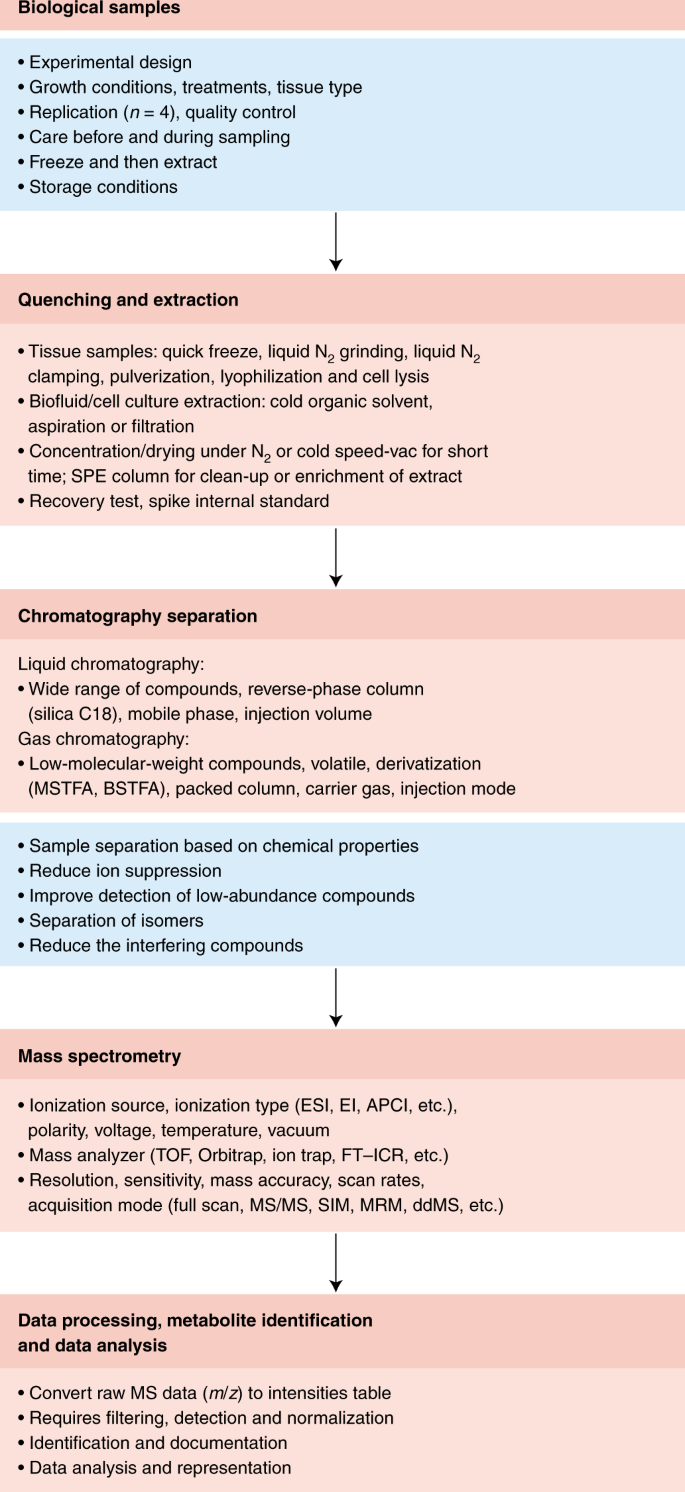
Overview chart listing the major steps and guidelines involved in typical MS-based metabolomics studies.
Quenching needs to satisfy two criteria: it should (1) completely terminate all enzyme and chemical activities and (2) avoid the perturbation of existing metabolite levels during harvesting. Details regarding specific considerations that need to be taken into account for quenching the metabolism of various species are provided in Supplementary Note 4 . The efficiency of quenching can be followed either by controlled comparisons of various extraction methods 38 or, alternatively, by determining the abundance of (stable isotope-labeled) standards spiked into the quenching solvent (see “Recovery and recombination experiments”). For tissues, where possible, quick excision followed by snap-freezing in liquid nitrogen is recommended, with subsequent storage of deep-frozen tissue at a constant −80 °C until the first application of extraction solvent. However, for bulky tissue, submersion in liquid nitrogen is not sufficient because the center of the tissue is cooled too slowly. In such cases, freeze-clamping, where tissue is almost instantaneously squashed flat between two prefrozen metal blocks (known as a Wohlehberger clamp), is preferred 39 , 50 .
Irrespective of the quenching method, the downstream steps of these processes also warrant caution. For example, improper freeze drying and lack of storage in sealed containers can generate artifactual geometric isomers of pigments 39 . Freeze drying is also unsuitable when volatile components are of interest. While the appropriate means of storage is strictly dependent on the stability of the class of targeted metabolites under study, it is not recommended to store samples between 0 and 40 °C. At these temperatures, substances can become concentrated in a residual aqueous phase 39 . It is therefore recommended, where necessary, to store completely dry residues for as short a time as possible before their analysis. In addition, great care must be taken to ensure that metabolism remains quenched during thawing. This is particularly pertinent for extracts containing secondary metabolites. In such extracts, degradative enzymes often retain their activities, which, if not kept in check, may result in the consumption or conversion of certain metabolites with a concomitant appearance of new compounds or breakdown products 51 .
Similar issues are also present with respect to both the experimental growth media and the initial extraction solvents used. Growth media often need to be removed via multiple wash steps to reduce the effects of ion suppression during the subsequent MS analysis, and the solvent used for initial extraction may need to be exchanged owing to incompatibility with the instrumentation used for the metabolite analysis. Two pitfalls are pertinent here: (1) the washing process results in the loss of metabolites and (2) solvent removal leads to concentration of the metabolites and thereby an acceleration of chemical reactions between them. Thus, considerable caution is advised in method optimization to ensure that extraction and handling methods allow adequate quantitative representation of cellular metabolites. In some instances, such as the analysis of volatile or semivolatile compounds, sample extraction and handling should only be performed on fresh material. We strongly recommend the adoption of recovery and recombination experiments (see below) when either a substantially novel metabolomics technique is introduced or a novel cell type, tissue or organism is studied.
Sample replication and randomization
An important issue is the nature and number of biological, technical and analytical replicates. Before using any new extraction protocol or analytical procedure and when working with new biological materials, it is essential to perform extensive pilot experiments to fully assess the technical variation that is necessary to design a statistically sound experiment. To avoid misunderstanding, we refer readers to the definitions of each type of replicate provided in ref. 39 . While analytical replicates, that is, replicates corresponding to repeated injection of the exact same extract, are useful in assessing machine performance, technical replicates, which encompass the entire experimental procedure, allow a far more comprehensive assessment of any experimental variance in data generation 39 . Indeed, such analyses are essential for the establishment of a new extraction or processing procedure or a new analytical technique as well as for the optimization of a new instrument.
Biological replication is even more important and should involve at least four but preferably more replicates; the required number of replicates depends on the desired statistical power, effect size and actual variance 52 . Care must be taken to acquire such replicates in a highly uniform manner. For plants, this can also mean collecting samples at the same time of day and under the same environmental conditions. In many instances, a full and independent repeat of a biological experiment is advisable 53 . There are different stages where technical replicates can be made: at sampling, quenching, extraction and analysis, replicates can be made independently of the entire process. In our experience, the extraction step is the most critical of these. Whether technical replication is needed in support of biological replication is highly dependent on the relative magnitudes of variation; in cases in which the biological variation greatly exceeds the technical variation, it is sensible to sacrifice the latter to increase the former. With new systems, pilot experiments are highly recommended to evaluate biological and technical variation and hence determine how many samples and how many replicates are needed to achieve statistical robustness 52 .
Careful spatiotemporal randomization of biological samples throughout a metabolomics experiment is equally essential. If a set of samples is analyzed in a nonrandom order, treatment and control samples or time points may end up being measured under very different conditions. As a result, interpretation can be confounded by sample age or shifting instrument performance, potentially occluding biological variation between sample groups or, worse, creating artifactual differences. This is particularly important in large-scale metabolic profiling studies to characterize the natural variation of metabolism, akin to genome-wide association studies 10 , 54 , 55 , 56 . In such experiments, weeks of instrument time may be required. Clear best-practice guidelines for such large-scale studies have been presented elsewhere 57 , 58 , 59 , 60 , so we will not discuss them further here.
Irrespective of the size of the experiment, the use of quality-control samples and batch correction is also essential 61 . Such experimental controls help monitor instrument performance and stability and, thereby, data quality. These controls ensure that missing data or peaks with low signal-to-noise ratios do not occur. Either mixtures of authenticated metabolite samples at defined concentrations or dry-stored aliquots of a broadly shared and appropriately standardized biological extract (for example, multi-kilogram extracts of Arabidopsis , E. coli , yeast or human cell lines) can serve as broadly useful reference samples. Use of these references enhances accurate quantification and makes it possible to more effectively use the data in metabolite databases 62 , 63 , 64 , 65 , 66 . A pooled quality-control sample allows for evaluation (and correction) of run order and batch effects within a study, but not necessarily across experiments, as is possible with reference material.
Quantification
The aforementioned details of extraction, storage and replication are equally applicable when ensuring the accuracy of any method of metabolite quantification, including those that target single metabolites (Fig. 2 ). The remainder of this article will address issues that are, at least partially, restricted to untargeted metabolomics approaches. There are several essential aspects requiring consideration here.
First, it is essential to ensure that the levels of all metabolites of potential interest can be detected and, ideally, can be measured within a linear range of detection. This is most readily achieved through analyses of independent dilutions of each extract. Additionally, for experiments that begin with intact tissues, it is important to ensure complete tissue disruption. In the case of cellular studies, one must further take into consideration whether to limit the study to the endogenous cellular metabolites or also assess the exometabolome. For these controls, and many others, we provide a list of reporting recommendations in the section below on transparency in measurement, metabolite annotation and documentation.
Metabolomics data are most frequently provided as relative quantities (that is, relative quantification is performed) with respect to a reference sample. This is in contrast to NMR-based studies, which usually provide absolute concentrations (that is, absolute quantification), with peak intensities directly proportional to concentrations and directly comparable across different peaks and samples. The relative intensities of LC–MS and GC–MS peaks representing different compounds do not directly correlate to absolute concentrations. This is due to the differential ionization efficiencies of the different metabolites within a complex mixture.
To address this issue, standard curves can be used to determine how signal intensity responds as a function of analyte concentration and, moreover, the range of linearity of this relationship 12 . The ability to generate such curves is of course dependent on the availability of validated pure standards. While relative values are highly useful in many contexts and indeed are the only way of expressing the levels and changes in level of non-annotated analytes, absolute values have much greater utility for determining enzyme binding site occupancies, the thermodynamics of metabolic reactions 12 , 67 and the molecular dynamics underlying the flow of atoms through a metabolic network 68 , 69 , 70 . A further advantage of the methods used for absolute quantification is that they can be readily adapted into a means of quality control for both quantification and the correctness of peak annotation, for example, through thermodynamics 71 . However, obtaining standard curves for thousands of metabolites in a complex mixture is currently not always practical. While many of the metabolite signals in such mixtures are nonlinear owing to a variety of reasons, including ion interaction, ion suppression, etc., which substantially complicates quantitation (as described in the next section), there are experimental tools allowing the extent of this problem to be quantified and reported. Quantification is particularly problematic in the case of external calibration, where quantification of standards is carried out in a far simpler mixture than that of the biological extract. Therefore, either internal quantification using isotopically labeled standards or quantification of a mixture of internal and external standards, as described below, is preferable.
A further aspect of quantification is the basis on which quantities are expressed for tissue samples. Data are often provided per gram of fresh or dry weight, while for body fluids they are often provided per volume. The case of cellular metabolomics is more complicated given that cell size is often variable; values are therefore typically provided per milligram of protein or based on cell counts. The basis on which both absolute and relative metabolite levels is provided is of fundamental importance—for example, values given on the basis of fresh weight can be dramatically influenced by the osmotic potential of the cell—yet is often not given enough consideration by the community.
Recovery and recombination experiments
Recovery experiments, in which authenticated standard compounds are added to the initial extraction solvent to assess losses during extraction, storage and handling, were vigorously championed in the 1970s to 1990s 72 and can provide persuasive evidence that the data reported are a valid reflection of cellular metabolite compositions 39 . Recent examples exist of validated methods in microbial, plant and mammalian systems 73 , 74 , 75 . However, the metabolomics community has been relatively slow in adopting these control procedures. This is partially explained by the lack of commercially available standards and/or simple synthetic approaches to make standards. Indeed, for unknown analytes, this approach is by its nature impossible.
Fortunately, there is an alternative approach—extract recombination—that circumvents this practical limitation. In this approach, the extract of a novel tissue is characterized by combination with that of a well-characterized reference material such as one from E. coli , Arabidopsis or human biofluids. Such experiments not only provide information concerning the appropriateness of the extraction buffer but additionally allow an assessment of so-called matrix effects caused by ion suppression 76 , 77 , 78 . These experiments additionally allow a quantitative assessment of the reliability of known peaks 79 . A schematic representation of recovery and metabolic recombination experiments is presented in Fig. 3 .
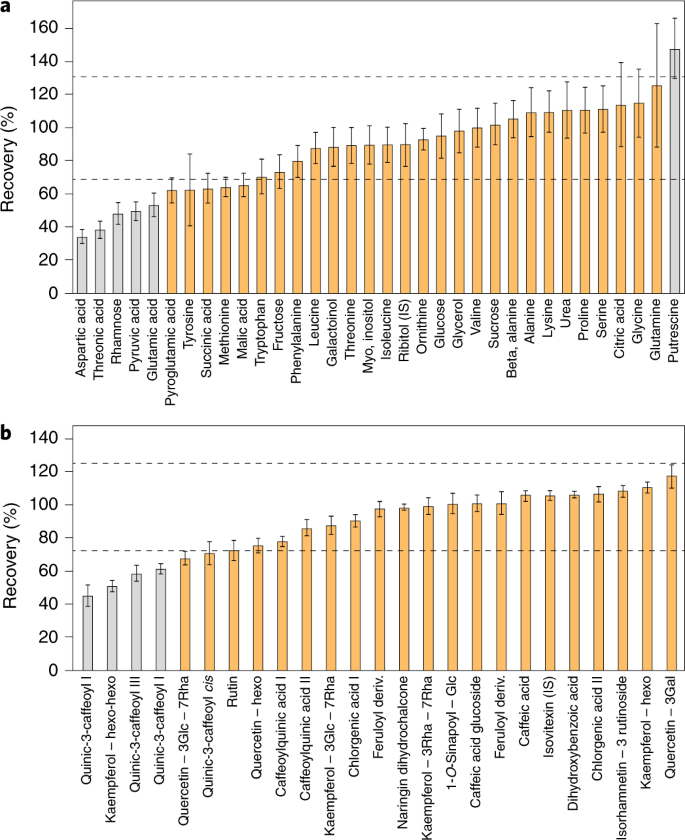
a , b , Recovery tests were performed using GC–MS ( a ) and LC–MS ( b ) peaks obtained for a mixture of extracts from Arabidopsis and lettuce leaves. The mixture was made by combining extracts from Arabidopsis (A) and lettuce (B) leaves (0.2 mg fresh weight per μl) at a 1:1 ratio. The percentage recovery was estimated using the theoretical concentration in the extract mixture: ((level in leaves (A) × A%) + (level in leaves (B) × B%))/100. Dashed lines indicate the acceptable range of 70–130%. Compounds in gray are statistically outside this range. Error bars represent ± s.e.m.
For known metabolites, we suggest that recovery or metabolic recombination experiments be carried out for each new tissue type or species. It is clear that in any metabolomics-scale study certain metabolites will have poor recovery. While this does not preclude the reporting of their values, it is important that this is documented to allow readers discretion in their interpretation. Recovery rates of 70–130% are acceptable, with anything deviating beyond this range representing a metabolite whose quantification should be subject to further testing. For example, even a 50% recovery rate—if reproducible and linear—could be deemed acceptable (Fig. 3 ). The importance of such control experiments is perhaps best illustrated by cases in which they were not performed. Anecdotally, there are several examples in the literature where the metabolite data reported cannot be reflective of cellular content, for example, because the zero levels reported for metabolites, if representative of cellular levels, would indicate that the cells tested were not viable.
Ion suppression
Despite the selectivity and sensitivity of MS techniques, there are considerable challenges with regard to reproducibility and accuracy when analyzing complex samples. These problems are not insurmountable but require that additional care be taken when interpreting results. Ion suppression is a general problem in LC–MS analyses due to matrix effects influencing the ionization of co-eluting analytes, affecting the precision and accuracy of quantification or preventing less abundant metabolites from being detected at all 76 , 78 , 80 . As mentioned above, the best method of assessing the potential impact of ion suppression is to mix two independent extracts in a recombination experiment (Fig. 3 ) and assess whether the metabolites detected can be quantitatively recovered 51 . Essentially, within this process, co-eluting analytes compete for the ionization energy, resulting in incomplete ionization. Therefore, a decreased ion count for an analyte may be due either to a decreased concentration of the analyte itself or to increased concentrations of co-eluting analytes. It is critically important to consider these effects during method validation to ensure the quality of the analysis.
While there is no universal solution to the ion suppression problem, assessing the effects of ion suppression affords greater confidence in the accuracy of the results. There are several strategies that can help minimize ion suppression 77 . Among these, improvements in sample preparation and chromatographic selectivity are currently the most effective. In some situations, using suitable clean-up procedures depending on sample type and analyte properties may allow removal of co-eluting components. This might involve simple dilution of extracts or the growth media from which the samples are derived 51 or optimization of various steps of sample work-up, including sonication, solvent partitioning, filtration, centrifugation and protein precipitation 81 . In addition, solid-phase extraction (SPE) using appropriate absorbents has been demonstrated to be an effective method to reduce matrix effects. Furthermore, it is possible to adjust chromatography conditions so that the peaks of interest do not elute in regions of suppression; for example, modifying the composition of the mobile phase or gradient conditions can aid chromatographic separation and thereby improve performance.
Careful selection of the ion source and column polarity is an alternative strategy to reduce ion suppression. For example, atmospheric pressure chemical ionization (APCI) is less prone to matrix effects than electrospray ionization (ESI). In addition, using APCI can also reduce interference effects 12 . It has been demonstrated that ion suppression is often less severe for negatively charged compounds than for positively charged ones 82 . Finally, although the above-mentioned strategies may not be sufficient to completely remove the effects of ion suppression in complex samples, the extent of the problem can at least be quantified by carrying out control experiments as described in the preceding section.
Peak misidentification
The orthogonal use of chromatography (either gas or liquid based) with MS and in some cases also tandem MS (MS/MS) fragmentation patterns provides great specificity 83 , 84 . Current high-end instruments detect on the order of 10,000 or 100,000 features; however, these include a large number of adduct and isotope peaks. Bioinformatics tools for analyte identification take this into account and even use commonly observed adducts as a means of identifying analytes (discussed in detail below). Nonetheless, there are three common problems that contribute to misidentification.
First, isomers—compounds with an identical molecular formula but distinct structures—are common in nature. Important examples from primary metabolism include hexose phosphates and inositol phosphates, citrate and isocitrate, glucose and fructose, and alanine and sarcosine. High-resolution MS alone may not suffice to discriminate between these and other sets of isomers, especially when fragmentation patterns are similar, and some types of isomers may not separate well on conventional reverse-phase high-performance LC (HPLC). To improve separation, reverse-phase ion pairing chromatography, hydrophilic interaction chromatography (HILIC) and other chromatographic methods can be used; another option is chemical derivatization before chromatography 12 . In cases where isomers cannot be separated, this needs to be clearly stated because such compounds may have greatly different biological functions.
Second, the presence of overlapping compounds may prevent detection of some metabolites. While the increasingly high resolution of mass spectrometers has mitigated this issue to some extent, the resolving power of many current instruments is insufficient to separate ions differing in mass by less than 5 parts per million (ppm) 12 . This problem, however, is only acute when chromatography is also unable to separate analytes that cannot be separated on the basis of mass.
The third major hurdle (which is more relevant for LC–MS than GC–MS) is the formation of in-source degradation products. These are by-product ions of ESI due to simple loss of water, carbon dioxide or hydrogen phosphate, more complicated molecular rearrangements and the attachment of other ions. In-source degradation reduces the intensity of the metabolite parent ion, and the resulting fragment ions may confound analysis of other co-eluting compounds, for example, if they have the same molecular formula as the molecular ion of another metabolite 12 . We provide examples of these from our own work in Supplementary Fig. 1 . These examples demonstrate the need for careful manual curation of all peak assignments, which, however, is often not feasible when annotating several hundred or thousand metabolites (Fig. 4 ). In ambiguous cases, the exact identification of a peak can often be best demonstrated via comparative biochemical approaches, for example, by analyzing the metabolome in known mutants that can be anticipated to lack certain metabolites 24 , 85 or incubation of a purified peak with known enzymes or chemical treatments 73 . These methods can also be combined with other approaches such as using authenticated standards for isomer annotation 86 and dual-labeling approaches 87 .
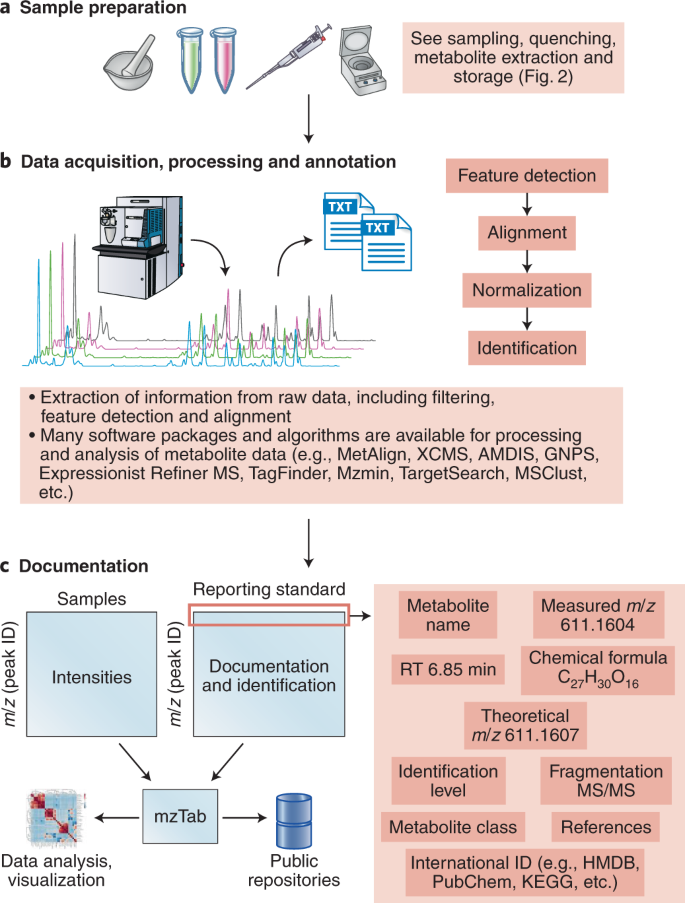
a , b , Structure elucidation workflow for data acquisition ( a ) and processing and annotation ( b ). c , Simple design for metabolic data documentation and how data can be linked to the mzTab 49 tool to facilitate data representation, sharing and deposition to public repositories.
As an aside, a critical aspect of nontargeted metabolomics is peak filtering. Metabolomics datasets from such studies contain a large proportion of uninformative features that can impede subsequent statistical analysis, and there is thus a need for versatile and data-adaptive methods for filtering data before investigating the underlying biological phenomena 88 . A list of suggestions for the design and implementation of data filtering strategies is provided in Supplementary Note 5 .
Reporting transparency
To fully exploit metabolomics data, they need to be comparable between different laboratories. Indeed, several comparative studies have been published, as we detail in Supplementary Note 6 . In addition to comparability at a quantitative level, clear metabolite ontologies are also needed to ensure that metabolites are annotated in a common fashion (Supplementary Note 7 ).
To ensure that methods can be readily adopted by others, a wealth of detailed information is required. However, detailed descriptions of sample preparation and analytical procedures are often (at least partially) absent in publications, especially in cases where metabolomics is not the primary focus of the published work. We recommend that the following items be considered as mandatory components of any methods section for metabolomics experiments.
Chromatography: composition of the mobile phase, column properties, temperature, flow rate and injection volume
Mass spectrometry: ionization source and type of detection mode, MS method, scan number and speed, and MS/MS parameters, including resolution settings and the energy used for fragmentation (Box 1 )
Extensive recommendations have been made before 36 , 39 ; however, we believe that this list will need to be revisited frequently owing to improvements in instrumentation and other aspects of the metabolomics workflow. If unsure of how much methodological detail to provide, imagine that your twin is sitting on a different continent in front of similar instrumentation and has to configure the equipment in a comparable manner. Increasingly, there is software support to extract such information from raw data files converted into, for example, the mzML file format 44 (Fig. 4c ).
Considering the number of possible pitfalls in the annotation and quantification of metabolites in metabolomics approaches, the current general level of reporting in the literature is not entirely satisfactory (Figs. 4 and 5 ). Given restrictive journal word limits and the fact that scientific reports tend to be highly concise, it is perhaps not surprising that authors do not refer to compounds as ‘the metabolite that we putatively annotate as X’ within the text of their articles. That said, there is nothing to preclude highly detailed reporting of the exact nature of the annotation within the supplementary data associated with a paper, either copublished or made available through separate web resources. Databases such as MetaboLights 89 and the Metabolomics Workbench 90 can be used for this purpose and indeed have been adopted as a requirement for many journals.
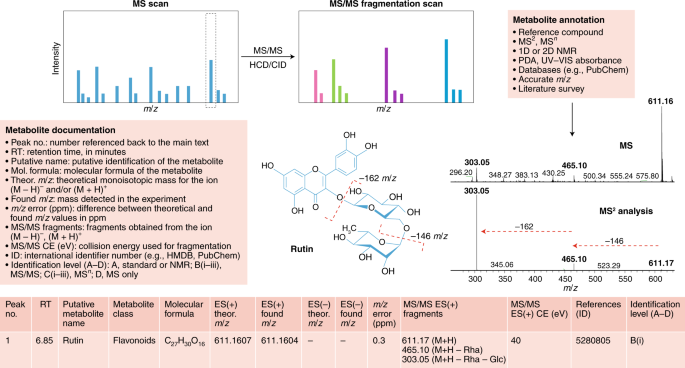
Structure elucidation workflow of metabolite identification. MS/MS fragmentation provides information about compound structure. Metabolite annotation can be achieved using reference compounds, MS 2 analysis, NMR or a photodiode array (PDA) detector for UV–visible light spectrum detection. Database searching enables molecular formula calculation. Illustrated is an example of our recommendations for reporting metabolomics data for a typical LC–MS experiment for the compound rutin (a flavonoid glycoside). Comparison of the MS and MS/MS spectra for rutin reveals a peak at 611 m / z in the MS scan and two major fragments at 611 m / z in the MS/MS scan, providing information about chemical loss of rhamnose (−146 m / z ) and glucose (–162 m / z ) moieties. For metabolite documentation, the current general recommended levels of reporting are shown; see Supplementary Tables 1 and 2 for further details.
We recommend a streamlined, simpler reporting approach (Fig. 5 ). While this is similar to that previously suggested for plant analyses 39 , we have updated reporting recommendations to ensure broader applicability and relevance. To simplify the adoption of these recommendations, we supply Supplementary Tables 1 and 2 as template Microsoft Excel spreadsheets. Supplementary Table 1 contains a list of simple questions regarding the reporting of metabolite data, and Supplementary Table 2 provides recommendations for metabolite annotation for typical GC–MS or LC–MS experiments. Once one is used to filling out these tables, it is our experience that it takes between 30 and 60 minutes to complete the process. In the case of large datasets consisting of hundreds to thousands of samples, which nowadays represent what is reported in a sizeable proportion of metabolomics papers, the time for upload in metabolomics repositories is thus considerably longer than the filling out of our suggested Excel tables.
Box 1 Information required for transparency in measurement and metabolite annotation and documentation
Chromatography
• Instrument description: manufacturer, model number, software and version 36 , 39
• Separation conditions: column parameters (model, number, thickness, diameter, length and particle size)
• Separation method: mobile-phase composition and modifiers, flow rate, gradient program, column temperature, pressure, temperature and injection: split or splitless and injection cycle time
• Instrument type and parameters: model, software and version 36 , 39
• Type of ionization: ESI, EI, APCI or others; positive or negative polarity; and other ionization parameters (voltage, gas, vacuum and temperature)
• Mass analyzer: TOF, Orbitrap, ion trap, FT-ICR, etc.; hybrid or single-mass analyzer used for the experiment; and collision energy used for fragmentation
• Instrument performance: resolution, sensitivity, mass accuracy and scan rates
• Acquisition mode: full scan, MSMS, SIM, MRM, ddMS, etc.
Metabolite documentation (minimum ontology)
• Details are presented in Fig. 4 and Supplementary Tables 1 and 2 . Included minimum proposed reporting data: retention time, theoretical monoisotopic mass, m / z detected in the experiment for (M − H) − and/or (M + H) + ions, m / z error (in ppm), MS/MS fragments obtained from the (M − H) − and/or (M + H) + ions, metabolite name and compound class 36 , 92
• For known compounds, we propose to add international identifiers (such as from HMDB, KEGG, PubChem, KNApSAcK, etc.)
• Quantified data, including peak intensity and area, etc., across the experiment must be provided in an .xls or .text file as a supplementary file
• Representative chromatogram(s) should be included to allow the assessment of metabolite identification
More extensive ontology
• Check requirements for repository submission 35
• Format data using formats such as NetCDF for MS data 93
• Include international metabolite identifiers
• State data availability: freely available, published or not
• Provide a summary of the experiment
• Indicate whether authenticated or reference spectra were used for identification
• Give details on code or other information used for analysis if available
• In the case of submission of downstream data (results), the minimum structure for table format and the experiment must be provided; see Hoffmann et al. 44 for an example
• In the case of submission of data to GNPS for molecular networking, see Jarmusch et al. 45 for an example
These represent recommendation in cases where the raw data or downstream results are submitted to repository databases (for example, MetaboLights, the Metabolomics Workbench, MetaPhen, GNPS, etc.)
In summary, we have presented here recommendations to improve the quality and cross-laboratory comparability of metabolic datasets. These range from recommendations on sampling and metabolite extraction, quantification and peak identification to guidelines on transparency in measurement and documentation, for which a data- rather than chromatogram-centric approach is suggested. We anticipate that the adoption of these recommendations will offer several advantages: (1) perusal of reported metadata will provide readers with the ability to assess the quality of the data reported and, as such, allow greater confidence in the conclusions drawn; (2) researchers will have a simple route to gain information needed to aid them in annotating their own experimental output and (3) data obtained by multiple laboratories may be compared more easily.
A recent example of comprehensive documentation of a metabolomics experiment is provided by the study of Price et al. 91 , who evaluated metabolite levels in understudied crop species, assembling an extensive database of the underlying data. Greater adoption of simple reporting tables such as the ones we describe here (Supplementary Tables 1 and 2 ) or the similar one proposed by Dorrestein and coworkers (for a comparison of these tables, see Supplementary Note 8 ) has the potential to elucidate general aspects of the metabolic response.
We would like to stress that the intention of the recommendations presented here is to encourage fuller and more faithful reporting of both metabolite annotations and their respective quantification. Our proposed reporting standards are not meant to be a direct replacement for the standards set by metabolome repositories. In fact, in most instances, these are entirely complementary to one another. We recommend that metabolomics practitioners follow repository standards alongside those we discuss here. There is a wealth of data reported in the literature that, for one reason or another, have not been deposited in repositories (such as MetaboLights, the Metabolomics Workbench and GNPS-MassIVE), and for such data it would be excellent if the metadata could be captured. This is important not only for possible reuse of the data but equally as a means of allowing the reader the possibility to evaluate their veracity. Expansion of such approaches, including input from both experimental and computational scientists, will facilitate the generation of pan-metabolome databases, which will undoubtedly open new horizons for metabolomics in all kingdoms of life.
We believe that more widespread adoption of these recommendations will enhance the quality of reporting of metabolite data, advance community efforts to improve the annotation of metabolomes and, finally, facilitate the exchange and comparability of metabolite data from different laboratories. These efforts will also facilitate comparison of metabolomics datasets obtained from different species, supporting the renaissance of comparative biochemistry.
Doerr, A. Global metabolomics. Nat. Methods 14 , 32 (2017).
Article CAS Google Scholar
Fessenden, M. Metabolomics: small molecules, single cells. Nature 540 , 153–155 (2016).
Article CAS PubMed Google Scholar
Oliver, S. G., Winson, M. K., Kell, D. B. & Baganz, F. Systematic functional analysis of the yeast genome. Trends Biotechnol. 16 , 373–378 (1998).
Alseekh, S. & Fernie, A. R. Metabolomics 20 years on: what have we learned and what hurdles remain? Plant J. 94 , 933–942 (2018).
Chevalier, C. et al. Gut microbiota orchestrates energy homeostasis during cold. Cell 163 , 1360–1374 (2015). This paper demonstrates that the microbiota is a key factor orchestrating overall energy homeostasis during increased demand in mammals .
Chu, C. et al. The microbiota regulate neuronal function and fear extinction learning. Nature 574 , 543–548 (2019).
Article CAS PubMed PubMed Central Google Scholar
Djamei, A. et al. Metabolic priming by a secreted fungal effector. Nature 478 , 395–398 (2011).
Dorr, J. R. et al. Synthetic lethal metabolic targeting of cellular senescence in cancer therapy. Nature 501 , 421–425 (2013). This paper illustrates the identification of metabolite biomarkers for use in cancer diagnostics and to serve as targets for new-concept anticancer therapies .
Article PubMed CAS Google Scholar
Fernie, A. R., Trethewey, R. N., Krotzky, A. J. & Willmitzer, L. Metabolite profiling: from diagnostics to systems biology. Nat. Rev. Mol. Cell Biol. 5 , 763–769 (2004).
Gieger, C. et al. Genetics meets metabolomics: a genome-wide association study of metabolite profiles in human serum. PLoS Genet. 4 , e100282 (2008).
Guijas, C., Montenegro-Burke, J. R., Warth, B., Spilker, M. E. & Siuzdak, G. Metabolomics activity screening for identifying metabolites that modulate phenotype. Nat. Biotechnol. 36 , 316–320 (2018).
Lu, W. et al. Metabolite measurement: pitfalls to avoid and practices to follow. Annu. Rev. Biochem. 86 , 277–304 (2017). A useful and comprehensive review highlighting the pitfalls encountered in metabolomics and providing guidelines for accurate metabolite measurements .
Mashego, M. R. et al. Microbial metabolomics: past, present and future methodologies. Biotechnol. Lett. 29 , 1–16 (2007).
Rinschen, M. M., Ivanisevic, J., Giera, M. & Siuzdak, G. Identification of bioactive metabolites using activity metabolomics. Nat. Rev. Mol. Cell Biol. 20 , 353–367 (2019).
Van Gulik, W. M. et al. Fast sampling of the cellular metabolome. Methods Mol. Biol. 881 , 279–306 (2012).
Delzenne, N. M. & Bindels, L. B. Microbiome metabolomics reveals new drivers of human liver steatosis. Nat. Med. 24 , 906–907 (2018).
Guo, A. C. et al. ECMDB: the E. coli metabolome database. Nucleic Acids Res. 41 , D625–D630 (2013).
Sajed, T. et al. ECMDB 2.0: a richer resource for understanding the biochemistry of E. coli . Nucleic Acids Res. 44 , D495–D501 (2016).
Hautbergue, T., Jamin, E. L., Debrauwer, L., Puel, O. & Oswald, I. P. From genomics to metabolomics, moving toward an integrated strategy for the discovery of fungal secondary metabolites. Nat. Prod. Rep. 35 , 147–173 (2018).
Ramirez-Gaona, M. et al. YMDB 2.0: a significantly expanded version of the yeast metabolome database. Nucleic Acids Res. 45 , D440–D445 (2017).
Wishart, D. S. et al. HMDB 4.0: the human metabolome database for 2018. Nucleic Acids Res. 46 , D608–D617 (2018). The database described is a groundbreaking, comprehensive and freely available web resource containing detailed information about the human metabolome .
Saito, K. & Matsuda, F. Metabolomics for functional genomics, systems biology, and biotechnology. Annu. Rev. Plant Biol. 61 , 463–489 (2010).
Lisec, J., Schauer, N., Kopka, J., Willmitzer, L. & Fernie, A. R. Gas chromatography mass spectrometry-based metabolite profiling in plants. Nat. Protoc. 1 , 387–396 (2006).
Tohge, T. & Fernie, A. R. Combining genetic diversity, informatics and metabolomics to facilitate annotation of plant gene function. Nat. Protoc. 5 , 1210–1227 (2010).
van Gulik, W. M. Fast sampling for quantitative microbial metabolomics. Curr. Opin. Biotechnol. 21 , 27–34 (2010).
Vuckovic, D. Current trends and challenges in sample preparation for global metabolomics using liquid chromatography–mass spectrometry. Anal. Bioanal. Chem. 403 , 1523–1548 (2012).
Wang, Z., Gerstein, M. & Snyder, M. RNA-seq: a revolutionary tool for transcriptomics. Nat. Rev. Genet. 10 , 57–63 (2009).
Bennett, B. D., Yuan, J., Kimball, E. H. & Rabinowitz, J. D. Absolute quantitation of intracellular metabolite concentrations by an isotope ratio-based approach. Nat. Protoc. 3 , 1299–1311 (2008).
Li, B. et al. NOREVA: normalization and evaluation of MS-based metabolomics data. Nucleic Acids Res. 45 , W162–W170 (2017).
Fiehn, O. Metabolomics—the link between genotypes and phenotypes. Plant Mol. Biol. 48 , 155–171 (2002).
Papadimitropoulos, M. P., Vasilopoulou, C. G., Maga-Nteve, C. & Klapa, M. I. Untargeted GC–MS metabolomics. Methods Mol. Biol. 1738 , 133–147 (2018).
Fiehn, O. et al. The metabolomics Standards Initiative (MSI). Metabolomics 3 , 175–178 (2007). A brief report outlining the history, stature and intentions of MSI, an authorative standards initiative for metabolomics .
Haug, K. et al. MetaboLights—an open-access general-purpose repository for metabolomics studies and associated meta-data. Nucleic Acids Res. 41 , D781–D786 (2013).
Salek, R. M., Haug, K. & Steinbeck, C. Dissemination of metabolomics results: role of MetaboLights and COSMOS. GigaScience 2 , 8 (2013).
Article PubMed PubMed Central Google Scholar
Steinbeck, C. et al. MetaboLights: towards a new COSMOS of metabolomics data management. Metabolomics 8 , 757–760 (2012).
Sumner, L. W. et al. Proposed minimum reporting standards for chemical analysis: Chemical Analysis Working Group (CAWG) Metabolomics Standards Initiative (MSI). Metabolomics 3 , 211–221 (2007).
Sansone, S. A. et al. The Metabolomics Standards Initiative. Nat. Biotechnol. 25 , 846–848 (2007). This article highlights two standards and guidelines papers for MS and sample preparation by the Human Proteome Organization Proteomics Standardization Initiative (HUPO-PSI) and the Functional Genomics Experiment (FuGE) and describes how metabolomics standards should align to these .
Spicer, R. A., Salek, R. & Steinbeck, C. A decade after the Metabolomics Standards Initiative: it’s time for a revision. Sci. Data 4 , 3 (2017).
Article Google Scholar
Fernie, A. R. et al. Recommendations for reporting metabolite data. Plant Cell 23 , 2477–2482 (2011).
Aksenov, A. A. et al. A machine learning workflow enables automatic deconvolution of GC-MS data. Nat. Biotechnol. 39 , 169–173 (2021).
Aron, A. T. et al. Reproducible molecular networking of untargeted mass spectrometry data using GNPS. Nat. Protoc. 15 , 1954–1991 (2020).
Blaženović, I. et al. Structure annotation of all mass spectra in untargeted metabolomics. Anal. Chem. 91 , 2155–2162 (2019).
Buendia, P. et al. Ontology-based metabolomics data integration with quality control. Bioanalysis 11 , 1139–1155 (2019).
Hoffmann, N. et al. mzTab-M: a data standard for sharing quantitative results in mass spectrometry metabolomics. Anal. Chem. 91 , 3302–3310 (2019).
Jarmusch, A. K. et al. ReDU: a framework to find and reanalyze public mass spectrometry data. Nat. Methods 17 , 901–904 (2020). This tool enables the capture of public MS-based metabolomics data and their subsequent reanalysis .
Nothias, L. F. et al. Feature-based molecular networking in the GNPS analysis environment. Nat. Methods 17 , 905–908 (2020).
Tsugawa, H. et al. A cheminformatics approach to characterize metabolomes in stable-isotope-labeled organisms. Nat. Methods 16 , 295–298 (2019).
Huan, T. et al. Systems biology guided by XCMS Online metabolomics. Nat. Methods 14 , 461–462 (2017).
Patel, V. R., Eckel-Mahan, K., Sassone-Corsi, P. & Baldi, P. CircadiOmics: integrating circadian genomics, transcriptomics, proteomics and metabolomics. Nat. Methods 9 , 772–773 (2012).
Palladino, G. W., Wood, J. J. & Proctor, H. J. Modified freeze clamp technique for tissue assay. J. Surg. Res. 28 , 188–190 (1980).
Tohge, T. et al. From models to crop species: caveats and solutions for translational metabolomics. Front. Plant Sci. 2 , 61 (2011).
Trutschel, D., Schmidt, S., Grosse, I. & Neumann, S. Experiment design beyond gut feeling: statistical tests and power to detect differential metabolites in mass spectrometry data. Metabolomics 11 , 851–860 (2015).
Sanchez, D. H., Szymanski, J., Erban, A., Udvardi, M. K. & Kopka, J. Mining for robust transcriptional and metabolic responses to long-term salt stress: a case study on the model legume Lotus japonicus . Plant Cell Environ. 33 , 468–480 (2010).
Chen, W. et al. Comparative and parallel genome-wide association studies for metabolic and agronomic traits in cereals. Nat. Commun. 7 , 12767 (2016).
Fuhrer, T., Zampieri, M., Sevin, D. C., Sauer, U. & Zamboni, N. Genomewide landscape of gene–metabolome associations in Escherichia coli . Mol. Syst. Biol. 13 , 907 (2017).
Article PubMed PubMed Central CAS Google Scholar
Hartiala, J. A. et al. Genome-wide association study and targeted metabolomics identifies sex-specific association of CPS1 with coronary artery disease. Nat. Commun. 7 , 10558 (2016).
Alseekh, S., Wu, S., Brotman, Y. & Fernie, A. R. Guidelines for sample normalization to minimize batch variation for large-scale metabolic profiling of plant natural genetic variance. Methods Mol. Biol. 1778 , 33–46 (2018).
Dunn, W. B. et al. Procedures for large-scale metabolic profiling of serum and plasma using gas chromatography and liquid chromatography coupled to mass spectrometry. Nat. Protoc. 6 , 1060–1083 (2011).
Griffin, J. L. et al. Standard reporting requirements for biological samples in metabolomics experiments: mammalian/in vivo experiments. Metabolomics 3 , 179–188 (2007).
Vandeputte, D., Tito, R. Y., Vanleeuwen, R., Falony, G. & Raes, J. Practical considerations for large-scale gut microbiome studies. FEMS Microbiol. Rev. 41 , S154–S167 (2017).
Wehrens, R. et al. Improved batch correction in untargeted MS-based metabolomics. Metabolomics 12 , 88 (2016).
Cui, Q. et al. Metabolite identification via the Madison Metabolomics Consortium Database. Nat. Biotechnol. 26 , 162–164 (2008).
Nakamura, Y. et al. KNApSAcK Metabolite Activity Database for retrieving the relationships between metabolites and biological activities. Plant Cell Physiol. 55 , e7 (2014).
Tautenhahn, R. et al. An accelerated workflow for untargeted metabolomics using the METLIN database. Nat. Biotechnol. 30 , 826–828 (2012).
Vinaixa, M. et al. Mass spectral databases for LC/MS- and GC/MS-based metabolomics: state of the field and future prospects. Trac-Trends Anal. Chem. 78 , 23–35 (2016).
Zhu, Z. J. et al. Liquid chromatography quadrupole time-of-flight mass spectrometry characterization of metabolites guided by the METLIN database. Nat. Protoc. 8 , 451–460 (2013).
Niebel, B., Leupold, S. & Heinemann, M. An upper limit on Gibbs energy dissipation governs cellular metabolism. Nat. Metab. 1 , 125–132 (2019).
Jourdan, F., Breitling, R., Barrett, M. P. & Gilbert, D. MetaNetter: inference and visualization of high-resolution metabolomic networks. Bioinformatics 24 , 143–145 (2008).
Pirhaji, L. et al. Revealing disease-associated pathways by network integration of untargeted metabolomics. Nat. Methods 13 , 770–776 (2016).
Shen, X. T. et al. Metabolic reaction network-based recursive metabolite annotation for untargeted metabolomics. Nat. Commun. 10 , 1516 (2019). This represents an important example of a tool using a metabolic reaction network that expands metabolite annotations without the need for a comprehensive standard spectral library .
Kummel, A., Panke, S. & Heinemann, M. Putative regulatory sites unraveled by network-embedded thermodynamic analysis of metabolome data. Mol. Syst. Biol. 2 , 2006.0034 (2006).
Ap Rees, T. & Hill, S. A. Metabolic control analysis of plant metabolism. Plant Cell Environ. 17 , 587–599 (1994).
Arrivault, S. et al. Use of reverse-phase liquid chromatography, linked to tandem mass spectrometry, to profile the Calvin cycle and other metabolic intermediates in Arabidopsis rosettes at different carbon dioxide concentrations. Plant J. 59 , 826–839 (2009).
Lu, W., Bennett, B. D. & Rabinowitz, J. D. Analytical strategies for LC–MS-based targeted metabolomics. J. Chromatogr. B Anal. Technol. Biomed. Life Sci. 871 , 236–242 (2008).
Lunn, J. E. et al. Sugar-induced increases in trehalose 6-phosphate are correlated with redox activation of ADP-glucose pyrophosphorylase and higher rates of starch synthesis in Arabidopsis thaliana . Biochem. J. 397 , 139–148 (2006).
Annesley, T. M. Ion suppression in mass spectrometry. Clin. Chem. 49 , 1041–1044 (2003).
Antignac, J. P., Marchand, P., Le Bizec, B. & Andre, F. Identification of ractopamine residues in tissue and urine samples at ultra-trace level using liquid chromatography–positive electrospray tandem mass spectrometry. J. Chromatogr. B Anal. Technol. Biomed. Life Sci. 774 , 59–66 (2002).
Kebarle, P. & Tang, L. From ions in solution to ions in the gas phase—the mechanism of electrospray mass spectrometry. Anal. Chem. 65 , 972A–986A (1993).
CAS Google Scholar
Roessner-Tunali, U. et al. De novo amino acid biosynthesis in potato tubers is regulated by sucrose levels. Plant Physiol. 133 , 683–692 (2003).
Buhrman, D. L., Price, P. I. & Rudewiczcor, P. J. Quantitation of SR 27417 in human plasma using electrospray liquid chromatography–tandem mass spectrometry: a study of ion suppression. J. Am. Soc. Mass Spectrom. 7 , 1099–1105 (1996).
Gerssen, A. et al. Solid phase extraction for removal of matrix effects in lipophilic marine toxin analysis by liquid chromatography–tandem mass spectrometry. Anal. Bioanal. Chem. 394 , 1213–1226 (2009).
Freitas, L. G., Götz, C. W., Ruff, M., Singer, H. P. & Müller, S. R. Quantification of the new triketone herbicides, sulcotrione and mesotrione, and other important herbicides and metabolites, at the ng/l level in surface waters using liquid chromatography–tandem mass spectrometry. J. Chromatogr. A 1028 , 277–286 (2004).
De Vijlder, T. et al. A tutorial in small molecule identification via electrospray ionization–mass spectrometry: the practical art of structural elucidation. Mass Spectrom. Rev. 37 , 607–629 (2018).
Dettmer, K., Aronov, P. A. & Hammock, B. D. Mass spectrometry-based metabolomics. Mass Spectrom. Rev. 26 , 51–78 (2007).
Tohge, T. et al. Functional genomics by integrated analysis of metabolome and transcriptome of Arabidopsis plants over-expressing an MYB transcription factor. Plant J. 42 , 218–235 (2005).
Shahaf, N. et al. The WEIZMASS spectral library for high-confidence metabolite identification. Nat. Commun. 7 , 12423 (2016). This represents a reference metabolite spectral library developed from high-resolution MS data acquired from a structurally diverse set of 3,540 plant metabolites, providing great promise for addressing the question of comprehensivity in metabolomics .
Feldberg, L., Venger, I., Malitsky, S., Rogachev, I. & Aharoni, A. Dual labeling of metabolites for metabolome analysis (DLEMMA): a new approach for the identification and relative quantification of metabolites by means of dual isotope labeling and liquid chromatography–mass spectrometry. Anal. Chem. 81 , 9257–9266 (2009).
Schiffman, C. et al. Filtering procedures for untargeted LC–MS metabolomics data. BMC Bioinformatics 20 , 334 (2019).
Kale, N. S. et al. MetaboLights: an open-access database repository for metabolomics data. Curr. Protoc. Bioinformatics 53 , 14.13.11–14.13.18 (2016).
Sud, M. et al. Metabolomics Workbench: an international repository for metabolomics data and metadata, metabolite standards, protocols, tutorials and training, and analysis tools. Nucleic Acids Res. 44 , D463–D470 (2016).
Price, E. J. et al. Metabolite database for root, tuber, and banana crops to facilitate modern breeding in understudied crops. Plant J. 101 , 1258–1268 (2020). Recent exemplary documentation of a metabolomics experiment that evaluated metabolite levels in crop species, providing not only an extensive database but moreover an excellent example of how to correctly investigate understudied species .
Bino, R. J. et al. Potential of metabolomics as a functional genomics tool. Trends Plant Sci. 9 , 418–425 (2004).
Kirwan, J. A. et al. Preanalytical processing and biobanking procedures of biological samples for metabolomics research: a white paper, community perspective (for ‘Precision Medicine and Pharmacometabolomics Task Group’–The Metabolomics Society Initiative). Clin. Chem. 64 , 1158–1182 (2018).
Download references
Acknowledgements
A.R.F. and S.A. are supported by the European Union’s Horizon 2020 research and innovation program, under PlantaSYST (SGA-CSA no. 739582 under FPA no. 664620). J.E. and S.S. were supported by German Research Foundation (DFG) grant numbers 210879364 and 239748522. P.D.F. is grateful for funding from the CGIAR Research Program on Roots, Tubers and Bananas (RTB) and is supported by CGIAR Fund Donors and the Biotechnology and Biological Sciences Research Council OPTICAR Project (project BB/P001742/1). R.D.H. acknowledges receipt of the Nils Foss Food Excellence Prize, which funded his time spent on this initiative. W.W. is supported by the Huazhong Agricultural University Scientific & Technological Self-Innovation Foundation (program no. 2017RC002). K.C. and M.P.S. are supported by the NIH under grant numbers 5U54HG010426-03 and 1U2CCA233311-01. H.T. acknowledges financial support from the Shanghai Municipal Science and Technology Major Project (2017SHZDZX01) and the National Natural Science Foundation of China (31821002). G.X. is supported by the National Natural Science Foundation of China (21934006). T.T. was supported by JSPS KAKENHI grants-in-aid (19H03249 and 19K06723). G.S. is supported by the NIH under grant number R35GM130385.
Author information
Authors and affiliations.
Max Planck Institute of Molecular Plant Physiology, Potsdam-Golm, Germany
Saleh Alseekh, Leonardo Perez de Souza, Aleksandra Skirycz & Alisdair R. Fernie
Institute of Plants Systems Biology and Biotechnology, Plovdiv, Bulgaria
Saleh Alseekh & Alisdair R. Fernie
Department of Plant and Environmental Sciences, Weizmann Institute of Science, Rehovot, Israel
Asaph Aharoni
Department of Life Sciences, Ben Gurion University of the Negev, Beersheva, Israel
Yariv Brotman
Department of Genetics, Stanford University School of Medicine, Stanford, CA, USA
Kévin Contrepois, Michael P. Snyder & Si Wu
Leibniz Institute of Plant Genetics and Crop Plant Research (IPK), Gatersleben, Germany
John D’Auria
Department of Bioinformatics, University of Jena, Jena, Germany
Jan Ewald & Stefan Schuster
Interfaculty Institute of Cell Biology, Eberhard Karls University of Tuebingen, Tuebingen, Germany
Jennifer C. Ewald
Biological Sciences, Royal Holloway University of London, Egham, UK
Paul D. Fraser
Max Planck Institute for Biology of Ageing, Cologne, Germany
Patrick Giavalisco
BU Bioscience, Wageningen Research, Wageningen, the Netherlands
Robert D. Hall
Laboratory of Plant Physiology, Wageningen University, Wageningen, the Netherlands
Molecular Systems Biology, Groningen Biomolecular Sciences and Biotechnology Institute, University of Groningen, Groningen, the Netherlands
Matthias Heinemann
Max Planck Institute for Terrestrial Microbiology, Marburg, Germany
Hannes Link
College of Tropical Crops, Hainan University, Haikou, China
Bioinformatics and Scientific Data, Leibniz Institute for Plant Biochemistry, Halle, Germany
Steffen Neumann
BioInnovation Institute, Copenhagen, Denmark
Jens Nielsen
Department of Biology and Biological Engineering, Chalmers University of Technology, Gothenburg, Sweden
Plant Molecular Science Center, Chiba University, Chiba, Japan
Kazuki Saito
RIKEN Center for Sustainable Resource Science, Yokohama, Japan
Institute for Molecular Systems Biology, ETH Zurich, Zurich, Switzerland
Uwe Sauer & Nicola Zamboni
Boyce Thompson Institute and Department of Chemistry and Chemical Biology, Cornell University, Ithaca, NY, USA
Frank C. Schroeder & Aleksandra Skirycz
Center for Metabolomics and Mass Spectrometry, Scripps Research Institute, La Jolla, CA, USA
Gary Siuzdak
Department of Biochemistry and MU Metabolomics Center, University of Missouri, Columbia, MO, USA
Lloyd W. Sumner
State Key Laboratory of Genetic Engineering, Zhongshan Hospital and School of Life Sciences, Human Phenome Institute, Metabonomics and Systems Biology Laboratory at Shanghai International Centre for Molecular Phenomics, Fudan University, Shanghai, China
Department of Biological Science, Nara Institute of Science and Technology, Ikoma, Japan
Takayuki Tohge
Singapore Phenome Center, Lee Kong Chian School of Medicine, School of Biological Sciences, Nanyang Technological University, Nanyang, Singapore
Key Laboratory of Horticultural Plant Biology (MOE), College of Horticulture and Forestry Sciences, Huazhong Agricultural University, Wuhan, China
CAS Key Laboratory of Separation Science for Analytical Chemistry, Dalian Institute of Chemical Physics, Chinese Academy of Sciences, Dalian, China
You can also search for this author in PubMed Google Scholar
Contributions
A.R.F. and SA. wrote the manuscript with contributions and input from all authors. All authors read and approved the final manuscript.
Corresponding authors
Correspondence to Saleh Alseekh or Alisdair R. Fernie .
Ethics declarations
Competing interests.
The authors declare no competing interests.
Additional information
Peer review information Nature Methods thanks Justin van der Hooft and the other, anonymous, reviewer(s) for their contribution to the peer review of this work. Allison Doerr was the primary editor on this article and managed its editorial process and peer review in collaboration with the rest of the editorial team.
Publisher’s note Springer Nature remains neutral with regard to jurisdictional claims in published maps and institutional affiliations.
Supplementary information
Supplementary information.
Supplementary Notes 1–8 and Fig. 1.
Supplementary Table 1
Metabolite reporting checklist.
Supplementary Table 2
Metabolite annotation and documentation.
Rights and permissions
Reprints and permissions
About this article
Cite this article.
Alseekh, S., Aharoni, A., Brotman, Y. et al. Mass spectrometry-based metabolomics: a guide for annotation, quantification and best reporting practices. Nat Methods 18 , 747–756 (2021). https://doi.org/10.1038/s41592-021-01197-1
Download citation
Received : 02 April 2020
Accepted : 27 May 2021
Published : 08 July 2021
Issue Date : July 2021
DOI : https://doi.org/10.1038/s41592-021-01197-1
Share this article
Anyone you share the following link with will be able to read this content:
Sorry, a shareable link is not currently available for this article.
Provided by the Springer Nature SharedIt content-sharing initiative
This article is cited by
Lavandula angustifolia mill. for a suitable non-invasive treatment against fungal colonization on organic-media cultural heritage.
- M. C. Sorrentino
- S. Pacifico
Heritage Science (2024)
Quartet metabolite reference materials for inter-laboratory proficiency test and data integration of metabolomics profiling
- Naixin Zhang
- Qiaochu Chen
- Yuanting Zheng
Genome Biology (2024)
An assessment of AcquireX and Compound Discoverer software 3.3 for non-targeted metabolomics
- Bret Cooper
- Ronghui Yang
Scientific Reports (2024)
Plastid ancestors lacked a complete Entner-Doudoroff pathway, limiting plants to glycolysis and the pentose phosphate pathway
- Sonia E. Evans
- Anya E. Franks
- Michael A. Phillips
Nature Communications (2024)
Identifying key soil characteristics for Francisella tularensis classification with optimized Machine learning models
- Fareed Ahmad
- Kashif Javed
- Muhammad Zubair Shabbir
Quick links
- Explore articles by subject
- Guide to authors
- Editorial policies
Sign up for the Nature Briefing: Translational Research newsletter — top stories in biotechnology, drug discovery and pharma.

Thin-layer chromatographic (TLC) separations and bioassays of plant extracts to identify antimicrobial compounds
Affiliations.
- 1 Forage-Animal Production Research Unit, Agricultural Research Service, United States Department of Agriculture; [email protected].
- 2 Forage-Animal Production Research Unit, Agricultural Research Service, United States Department of Agriculture.
- PMID: 24747583
- PMCID: PMC4158999
- DOI: 10.3791/51411
A common screen for plant antimicrobial compounds consists of separating plant extracts by paper or thin-layer chromatography (PC or TLC), exposing the chromatograms to microbial suspensions (e.g. fungi or bacteria in broth or agar), allowing time for the microbes to grow in a humid environment, and visualizing zones with no microbial growth. The effectiveness of this screening method, known as bioautography, depends on both the quality of the chromatographic separation and the care taken with microbial culture conditions. This paper describes standard protocols for TLC and contact bioautography with a novel application to amino acid-fermenting bacteria. The extract is separated on flexible (aluminum-backed) silica TLC plates, and bands are visualized under ultraviolet (UV) light. Zones are cut out and incubated face down onto agar inoculated with the test microorganism. Inhibitory bands are visualized by staining the agar plates with tetrazolium red. The method is applied to the separation of red clover (Trifolium pratense cv. Kenland) phenolic compounds and their screening for activity against Clostridium sticklandii, a hyper ammonia-producing bacterium (HAB) that is native to the bovine rumen. The TLC methods apply to many types of plant extracts and other bacterial species (aerobic or anaerobic), as well as fungi, can be used as test organisms if culture conditions are modified to fit the growth requirements of the species.
Publication types
- Research Support, U.S. Gov't, Non-P.H.S.
- Video-Audio Media
- Anti-Infective Agents / isolation & purification*
- Anti-Infective Agents / pharmacology*
- Chromatography, Thin Layer / methods*
- Clostridium sticklandii / drug effects
- Microbial Sensitivity Tests
- Phenols / isolation & purification
- Phenols / pharmacology
- Plant Extracts / isolation & purification*
- Plant Extracts / pharmacology*
- Plants / chemistry*
- Rumen / microbiology
- Trifolium / chemistry
- Anti-Infective Agents
- Plant Extracts

An official website of the United States government
The .gov means it’s official. Federal government websites often end in .gov or .mil. Before sharing sensitive information, make sure you’re on a federal government site.
The site is secure. The https:// ensures that you are connecting to the official website and that any information you provide is encrypted and transmitted securely.
- Publications
- Account settings
Preview improvements coming to the PMC website in October 2024. Learn More or Try it out now .
- Advanced Search
- Journal List
- Plants (Basel)

Phytochemicals: Extraction, Isolation, and Identification of Bioactive Compounds from Plant Extracts
Ammar altemimi.
1 Department of Food Science, College of Agriculture, University of Al-Basrah, Basrah 61004, Iraq
Naoufal Lakhssassi
2 Department of Plant, Soil and Agricultural Systems, Plant Biotechnology and Genome Core-Facility, Southern Illinois University at Carbondale, Carbondale, IL 62901, USA; [email protected] (N.L.); ude.uis@ieuolrahab (A.B.); ude.uis@nostawd (D.G.W.); ude.uis@2804ag (D.A.L.)
Azam Baharlouei
Dennis g. watson, david a. lightfoot, associated data.
There are concerns about using synthetic phenolic antioxidants such as butylated hydroxytoluene (BHT) and butylated hydroxyanisole (BHA) as food additives because of the reported negative effects on human health. Thus, a replacement of these synthetics by antioxidant extractions from various foods has been proposed. More than 8000 different phenolic compounds have been characterized; fruits and vegetables are the prime sources of natural antioxidants. In order to extract, measure, and identify bioactive compounds from a wide variety of fruits and vegetables, researchers use multiple techniques and methods. This review includes a brief description of a wide range of different assays. The antioxidant, antimicrobial, and anticancer properties of phenolic natural products from fruits and vegetables are also discussed.
1. Introduction
Many antioxidant compounds can be found in fruits and vegetables including phenolics, carotenoids, anthocyanins, and tocopherols [ 1 ]. Approximately 20% of known plants have been used in pharmaceutical studies, impacting the healthcare system in positive ways such as treating cancer and harmful diseases [ 2 ]. Plants are able to produce a large number of diverse bioactive compounds. High concentrations of phytochemicals, which may protect against free radical damage, accumulate in fruits and vegetables [ 3 ]. Plants containing beneficial phytochemicals may supplement the needs of the human body by acting as natural antioxidants [ 4 ]. Various studies have shown that many plants are rich source of antioxidants. For instance, vitamins A, C, E, and phenolic compounds such as flavonoids, tannins, and lignins, found in plants, all act as antioxidants [ 3 ]. The consumption of fruits and vegetables has been linked with several health benefits, a result of medicinal properties and high nutritional value [ 5 ]. Antioxidants control and reduce the oxidative damage in foods by delaying or inhibiting oxidation caused by reactive oxygen species (ROS), ultimately increasing the shelf-life and quality of these foods [ 6 ]. Beta carotene, ascorbic acid, and many phenolics play dynamic roles in delaying aging, reducing inflammation, and preventing certain cancers [ 7 ]. Increasing the consumption of fruits and vegetables has been recommended by many agencies and health care systems throughout the world [ 8 ].
The objective of this paper is to provide a review of phytochemical studies that have addressed extracting, measuring and identifying bioactive compounds of plants. This review includes an overview of the lipid oxidation process, details of plants known to be antioxidant and antimicrobial sources, phenolic compounds, antioxidants from vegetables and fruits, cancer prevention, extraction techniques for phenolic compounds, isolation and purification of bioactive molecules, and techniques for structural classification of bioactive molecules.
2. Methods Used for Bioactive Compound Extraction, Isolation, and Purification
2.1. extraction of phenolic compounds using solvents.
Scientists have studied and analyzed the impact of different types of solvents, such as methanol, hexane, and ethyl alcohol, for the purpose of antioxidant extraction from various plants parts, such as leaves and seeds. In order to extract different phenolic compounds from plants with a high degree of accuracy, various solvents of differing polarities must be used [ 9 ]. Moreover, scientists have discovered that highly polar solvents, such as methanol, have a high effectiveness as antioxidants.
Anokwuru et al. reported that acetone and N , N dimethylformamide (DMF) are highly effective at extracting antioxidants, while Koffi et al. found that methanol was more effective in at a large amount of phenolic contents from walnut fruits when compared to ethanol [ 10 , 11 , 12 ].
It has been reported that ethanolic extracts of Ivorian plants extracted higher concentrations/amount of phenolics compared to acetone, water, and methanol [ 11 ]. Multiple solvents have been commonly used to extract phytochemicals, and scientists usually employed a dried powder of plants to extract bioactive compounds and eliminate the interference of water at the same time.
Solvents used for the extraction of biomolecules from plants are chosen based on the polarity of the solute of interest. A solvent of similar polarity to the solute will properly dissolve the solute. Multiple solvents can be used sequentially in order to limit the amount of analogous compounds in the desired yield. The polarity, from least polar to most polar, of a few common solvents is as follows: Hexane < Chloroform < Ethylacetate < Acetone < Methanol < Water.
2.2. Microwave-Assisted Extraction (MAE)
MAE has attracted the attention of researchers as a technique to extract bioactive compounds from a wide variety of plants and natural residues [ 12 ]. Microwaves have electromagnectic radiation that occurs at frequencies between 300 MHz to 300 GHz, and wavelengths between 1 cm and 1 m. These electromagnetic waves consist of both an electrical field and a magnetic field. These are described as two perpendicular fields. The first application of microwaves was to heat up objects that can absorb a part of the electromagnetic energy and convert it into heat. Commercial microwave instruments commonly use the frequency 2450 MHz, which corresponds to an energy output of 600–700 Watts [ 13 ].
Recently, advanced techniques have become available to reduce the loss of bioactive compound without increasing the extraction time. Therefore, microwave-assisted extraction is demonstrated to be a good technique in multiple fields, especially in the medicinal plant area. Moreover, this technique reduced the losses of the biochemical compounds being extracted [ 14 ]. Microwave-assisted extraction (MAE) has been used as an alternative to conventional techniques for the extraction of antioxidants because of its ability to reduce both time and extraction solvent volume [ 15 ]. In fact, the main objective of using MAE is to heat the solvent and extract antioxidants from plants with a lesser amount of these solvents [ 13 ].
Li et al. reported that conventional methods using various solvents presented less antioxidant activity and phenolic content than MAE [ 16 ]. Therefore, the finding confirmed that MAE was more effective at increasing antioxidant activity by measuring ferric reducing antioxidant power (FRAP), oxygen radical absorbance capacity (ORAC), and total phenolic content (TPC). The efficiency of the microwave extraction can be changed through some factors such as extraction temperature, solvent composition, and extraction time. The extraction temperature was usually studied more than other factors due to its ability to increase the efficiency of the microwave extraction. Tsubaki et al. reported that 170 °C was the most effective temperature for extracting phenolic compounds from Chinese tea. In addition, increasing the extraction temperature beyond this point resulted in a reduced extraction yield [ 17 ]. Recently, Christophoridou et al. used a new microwave-assisted extraction (MAE) process, which converts energy to heat, thereby cooperating with solvents in order to extract a specific compound [ 18 ]. Williams et al. showed many advantages of MAE, including lower solvent consumption, shorter extraction times, and higher sensitivity towards target molecules [ 19 ]. A comparison of some antioxidant methods used has been provided in Table 1 .
Comparison of methods for assessing antioxidant capacity based upon mechanism, endpoint, quantitation method, and whether the assay is adaptable to measure lipophilic and hydrophilic antioxidants.
2.3. Ultrasonic-Assisted Extraction
Ultrasound-assisted extraction (UAE) has been used in diverse applications of food-processing technology to extract bioactive compounds from plant materials [ 19 ]. Ultrasound, with levels greater than 20 kHz, is used to disrupt plant cell walls, which helps improve the solvent’s ability to penetrate the cells and obtain a higher extraction yield. UAE can use a low operating temperature through processing, maintaining a high extract quality for compounds. UAE is known to be one of the easiest extraction techniques because it uses common laboratory equipment such as an ultrasonic bath. In this technique, a smashed sample is mixed with the suitable solvent and placed into the ultrasonic bath, while temperature and extraction time are controlled [ 20 ].
UAE of various organic and inorganic samples can use a wide range of solvents. Common equipment used in ultrasound-assisted extraction includes an ultrasonic bath and an ultrasonic probe system. Unfortunately, ultrasonic probe has two main negative properties mainly related to experimental repeatability and reproducibility [ 21 ].
Tabaraki et al. noted that green technology is necessary to protect the environment from toxic substances [ 22 ]. Therefore, extraction of phenolic compounds by ultrasound has grown during recent years due to its role in reducing the amount of solvent and energy used. Corrales et al. have shown that UAE can break down plant tissue and work properly during the production process and release of active compounds in solvents with a high efficiency [ 21 ]. Results showed an increase in antioxidant activity from 187.13 μmol TE g −1 DM to 308 μmol TE g −1 DM by using UAE as an effective method to extract antioxidants from different sources. Recently, Albu et al. studied and applied the use of ultrasound to extract phenolic compounds from rosemary [ 23 ]. Multiple criteria have been compared including ultrasonic bath extractions, ultrasonic probe system, a shaking water bath at various temperatures, and different solvents to select the most efficient method. In all situations, the operation time was dramatically decreased by applying and using the ultrasonic bath and probe systems.
Similar behavior was reported by Cho et al. when extracting resveratrol from grapes [ 24 ]. In another study, Barbero et al. suggested the use of ultrasound in different industries because of its positive effects in the extraction of capsaicinoids of hot peppers [ 25 ]. Moreover, the ultrasonic method had the ability to decrease the degradation of phenolics [ 26 ]. Mulinacci et al. compared the extraction time of phenolic compounds from strawberries with other extraction methods such as solid–liquid, subcritical water, and microwave-assisted method [ 27 ]. The results confirmed that UAE was the most effective method.
2.4. Techniques of Isolation and Purification of Bioactive Molecules from Plants
Purification and isolation of bioactive compounds from plants is a technique that has undergone new development in recent years [ 28 , 29 ]. This modern technique offers the ability to parallel the development and availability of many advanced bioassays on the one hand, and provided precise techniques of isolation, separation, and purification on the other. The goal when searching for bioactive compounds is to find an appropriate method that can screen the source material for bioactivity such as antioxidant, antibacterial, or cytotoxicity, combined with simplicity, specificity, and speed [ 27 ].
In vitro methods are usually more desirable than in vivo assays because animal experiments are expensive, take more time, and are prone to ethical controversies. There are some factors that make it impossible to find final procedures or protocols to isolate and characterize certain bioactive molecules. This could be due to different parts (tissues) in a plant, many of which will produce quite different compounds, in addition to the diverse chemical structures and physicochemical properties of the bioactive phytochemicals [ 30 ]. Both the selection and the collection of plant materials are considered primary steps to isolate and characterize a bioactive phytochemical. The next step involves a retrieval of ethno-botanical information to discern possible bioactive molecules. Extracts can then be made with various solvents to isolate and purify the active compounds that are responsible for the bioactivity. Column chromatographic techniques can be used for the isolation and purification of the bioactive compounds. Developed instruments such as High Pressure Liquid Chromatography (HPLC) accelerate the process of purification of the bioactive molecule. Different varieties of spectroscopic techniques like UV-visible, Infrared (IR), Nuclear Magnetic Resonance (NMR), and mass spectroscopy can identify the purified compounds [ 31 ].
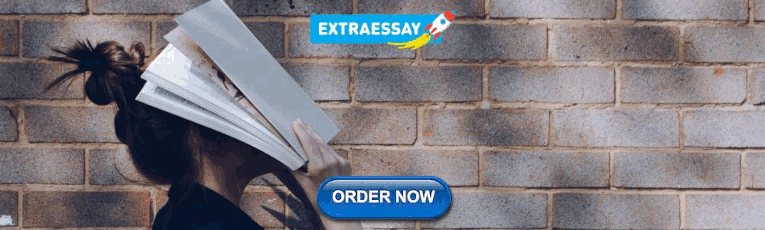
2.5. Purification of the Bioactive Molecule
Many bioactive molecules have been isolated and purified by using paper thin-layer and column chromatographic methods. Column chromatography and thin-layer chromatography (TLC) are still mostly used due to their convenience, economy, and availability in various stationary phases [ 32 ]. Silica, alumina, cellulose, and polyamide exhibit the most value for separating the phytochemicals. Plant materials include high amounts of complex phytochemicals, which make a good separation difficult [ 32 ]. Therefore, increasing polarity using multiple mobile phases is useful for highly valued separations. Thin-layer chromatography has always been used to analyze the fractions of compounds by column chromatography. Silica gel column chromatography and thin-layer chromatography (TLC) have been used for separation of bioactive molecules with some analytical tools [ 32 ].
2.6. Structural Clarification of the Bioactive Molecules
Determination of the structure of certain molecules uses data from a wide range of spectroscopic techniques such as UV-visible, Infrared (IR), Nuclear Magnetic Resonance (NMR), and mass spectroscopy. The basic principle of spectroscopy is passing electromagnetic radiation through an organic molecule that absorbs some of the radiation, but not all. By measuring the amount of absorption of electromagnetic radiation, a spectrum can be produced. The spectra are specific to certain bonds in a molecule. Depending on these spectra, the structure of the organic molecule can be identified. Scientists mainly use spectra produced from either three or four regions—Ultraviolet (UV), Visible, Infrared (IR), radio frequency, and electron beam [ 31 ]—for structural clarification.
2.7. UV-Visible Spectroscopy
UV-visible spectroscopy can be performed for qualitative analysis and for identification of certain classes of compounds in both pure and biological mixtures. Preferentially, UV-visible spectroscopy can be used for quantitative analysis because aromatic molecules are powerful chromophores in the UV range. Natural compounds can be determined by using UV-visible spectroscopy [ 33 ]. Phenolic compounds including anthocyanins, tannins, polymer dyes, and phenols form complexes with iron that have been detected by the ultraviolet/visible (UV-Vis) spectroscopy [ 34 ]. Moreover, spectroscopic UV-Vis techniques were found to be less selective and give information on the composition of the total polyphenol content. The UV-Vis spectroscopy was used to determine the total phenolic extract (280 nm), flavones (320 nm), phenolic acids (360 nm), and the total anthokyanids (520 nm). This technique is not time-consuming, and presents reduced cost compared to other techniques [ 35 ].
2.8. Infrared Spectroscopy
Some of the frequencies will be absorbed when infrared light passes through a sample of an organic compound; however, some frequencies will be transmitted through the sample without any absorption occurring. Infrared absorption is related to the vibrational changes that happen inside a molecule when it is exposed to infrared radiation. Therefore, infrared spectroscopy can essentially be described as a vibrational spectroscopy. Different bonds (C–C, C=C, C≡C, C–O, C=O, O–H, and N–H) have diverse vibrational frequencies. If these kinds of bonds are present in an organic molecule, they can be identified by detecting the characteristic frequency absorption band in the infrared spectrum [ 35 ]. Fourier Transform Infrared Spectroscopy (FTIR) is a high-resolution analytical tool to identify the chemical constituents and elucidate the structural compounds. FTIR offers a rapid and nondestructive investigation to fingerprint herbal extracts or powders.
2.9. Nuclear Magnetic Resonance Spectroscopy (NMR)
NMR is primarily related to the magnetic properties of certain atomic nuclei; notably the nucleus of the hydrogen atom, the proton, the carbon, and an isotope of carbon. NMR spectroscopy has enabled many researchers to study molecules by recording the differences between the various magnetic nuclei, and thereby giving a clear picture of what the positions of these nuclei are in the molecule. Moreover, it will demonstrate which atoms are present in neighboring groups. Ultimately, it can conclude how many atoms are present in each of these environments [ 33 ]. Several attempts have been made in the past by using preparative or semi preparative thin-layer chromatography, liquid chromatography, and column chromatography to isolate individual phenols, the structures of which are determined subsequently by NMR off-line [ 34 ].
2.10. Mass Spectrometry for Chemical Compounds Identification
Organic molecules are bombarded with either electrons or lasers in mass spectrometry and thereby converted to charged ions, which are highly energetic. A mass spectrum is a plot of the relative abundance of a fragmented ion against the ratio of mass/charge of these ions. Using mass spectrometry, relative molecular mass (molecular weight) can be determined with high accuracy and an exact molecular formula can be determined with a knowledge of places where the molecule has been fragmented [ 18 ]. In previous work, bioactive molecules from pith were isolated and purified by bioactivity-guided solvent extraction, column chromatography, and HPLC [ 36 ]. The techniques of UV-visible, IR, NMR, and mass spectroscopy were employed to characterize the structure of the bioactive molecule. Furthermore, molecules may be hydrolyzed and their derivatives characterized. Mass spectrometry provides abundant information for the structural elucidation of the compounds when tandem mass spectrometry (MS) is applied. Therefore, the combination of HPLC and MS facilitates rapid and accurate identification of chemical compounds in medicinal herbs, especially when a pure standard is unavailable [ 37 , 38 , 39 , 40 ]. Recently, LC/MS has been extensively used for the analysis of phenolic compounds. Electrospray ionization (ESI) is a preferred source due to its high ionization efficiency for phenolic compounds.
3. Lipid Oxidation
Lipid oxidation can occur during the processing, shipping, and storing of many foods. Lipids (such as triglycerides, sterols, and phospholipids) readily become oxidized with exposure to an oxidative environment [ 41 ]. Lipid molecules, especially those carrying polyunsaturated double bonds (i.e., linolenic acids), readily undergo oxidation within foods. Oxidatively stable oil with high melting temperature is necessary for solid fat application, and thus, highly saturated seed oil (palmitic acid and stearic acid) would be suitable for this end use [ 42 ]. Soybeans provide 56% of the world’s oilseed production. However, the percentage of saturated oil is very low in seed plants (about 10%), if compared to unsaturated oil (about 90%) [ 43 ]. Palmitic acid improves the oxidative stability of soybean oil, and can also be used to produce trans -fat-free shortening, margarine, and cosmetic products. However, this saturated short-chain fatty acid is undesirable for nutrition because its consumption results in an unfavorable lipoprotein profile in blood serum [ 44 ]. Stearic acid does not exhibit these cholesterolemic effects on human health [ 45 ]. Stearic acid is less likely to be incorporated into cholesterol esters and has a neutral effect on the concentration of blood serum LDL cholesterol [ 46 , 47 ]. Extensive research has been performed in order to increase stearic acid content oil production in the most widely consumed legume crop in the world, soybeans. By employing induced mutagenesis, seed stearic acid content was increased by up to 7 times [ 48 ].
Lipid oxidation in food systems can be caused by oxygen free radicals or reactive oxygen species. Free radicals are molecules with one or more unpaired electrons that work independently to cause oxidation [ 49 ]. Reactive oxygen species are a perfect example of oxygen free radicals. Reactive oxygen species do not solely contain free radical molecules, but also some non-free radicals that can influence lipid oxidation. Examples of non-free radical reactive oxygen species are hydrogen peroxide (H 2 O 2 ), hydrochloric acid (HCl), ozone (O 3 ), and molecular oxygen (O 2 ) [ 42 ]. Molecular oxygen can react with linoleic acid about 1450 times faster than triplet oxygen. One of the major causes of oil rancidity is molecular oxygen. Lipid oxidation caused by the chain reaction of free radicals can be illustrated in three stages: initiation, propagation, and termination [ 42 ]:
RH + initiator → R
ROOH + initiator → ROO•
R + O 2 → ROO
ROO + RH → ROOH + R•
R + R → R-R
ROO• + R → ROOH
The processes above occur in response to several physical or chemical factors including heating, radiation, temperature, metal ion catalysts, reactive oxygen species, and photosensitizers such as chlorophyll. The initiation step, shown in Equation (1), often happens at either an allytic methylene group of an unsaturated fatty acid (RH) or a lipid-hydroperoxide (ROOH). Next, the generated free radical (R•) reacts with oxygen to form a peroxy radical (ROO•). This product can directly react with another lipid molecule to produce a lipid hydroperoxide (ROOH), and thus a lipid free radical (R•). This causes continuously cascading chain reactions to occur until the free radicals are neutralized by other free radicals. This whole stage is shown in Equation (2). In the termination step, there are two radicals that have converted into non-free radical products, and thus will stop the cascade mode of the chain reaction according to Equation (3). Moreover, the reaction chain can also be terminated by some antioxidants or free radical scavengers. Metal ions, especially those of iron and copper, effectively catalyze these reactions [ 50 ].
Lipoxygenases (EC 1.13.11) can also act, causing oxidation to produce the peroxides in food materials that contain lipids. Hydrogen peroxide is one of the primary products of the oxidation, and it is very unstable and easily converts into secondary products. The final product of oxidation may include different chemical groups such as aldehydes, ketones, alcohols, acids, or hydrocarbons. These kinds of compounds can have a negative effect on the appearance, quality, and edibility of a food product by changing the texture, color, flavor, and safety of foods, or also by producing unacceptable off odors or off tastes, even negatively affecting the nutritional value [ 50 ].
4. Plants as a Source of Antioxidants
Antioxidants can be defined as bioactive compounds that inhibit or delay the oxidation of molecules [ 42 ]. Antioxidants are categorized as natural or synthetic antioxidants. Some synthetic antioxidants commonly used are: BHT, BHA, propyl gallate, and tertbutylhydroquinine. Many scientists have concerns about safety because synthetic antioxidants have recently been shown to cause health problems such as liver damage, due to their toxicity and carcinogenicity. Therefore, the development of safer antioxidants from natural sources has increased, and plants have been used as a good source of traditional medicines to treat different diseases. Many of these medicinal plants are indeed good sources of phytochemicals that possess antioxidant activities. Some typical examples of common ingredients that have been used in ethnic foods are tamarind, cardamom, lemon grass, and galangal basil. These spices or herbs have been shown to contain antioxidants [ 51 ].
Deterioration of food due to either bacterial or fungal infection has always been a major concern, causing huge losses to food industries and societies throughout the world [ 51 ]. Moreover, the spread of food pathogens has become a major public health concern. With an increasing awareness of the negative effects of synthetic preservatives, there has been increased demand for the use of nontoxic, natural preservatives, many of which are likely to have either antioxidant or antimicrobial activities [ 52 , 53 ]. Herbs have always been used for flavor and fragrance in the food industry, and some of them have been found to exhibit antimicrobial properties [ 54 ]. Therefore, the call for screening and using plant materials for their antioxidant and antimicrobial properties has increased. Approximately 20% of all plant species have been tested in both pharmacological and biological applications to confirm their safety and advantages [ 3 ]. A summary of the types of compounds, plant species, plant parts from which compounds were extracted, etc. can be found in Table S1 .
4.1. Presence of Antioxidant in Red Algae
Red algae are aquatic plant species considered one of the oldest groups of eukaryotic algae [ 55 ]. The antioxidant activity of a red alga, Palmaria palmate , has been studied. The results reported that 9.68 μg of ascorbic acid and 10.3 μg of total polyphenol can equally reduce activity in 1 mg of dulse extracts. The reducing activity was correlated with aqueous/alcohol soluble compounds due to the presence of functional groups such as hydroxyl, carbonyl, etc., which lead to reduced or inhibited oxidation [ 56 ].
4.2. Antioxidants from Monocots
Ashawat et al. studied the antioxidant properties of ethanolic extracts of Areca catechu and showed that Areca catechu had the highest antioxidant activity when compared to other eudicots like Centella asiatica, Punica granatum , and Glycyrrhiza glabra [ 57 ]. Londonkar and kamble studied Pandanus odoratissimus L. in order to determine its antioxidant activity [ 58 ]. Zahin et al. screened Acorus calamus to estimate antioxidant activity and total phenolic contents [ 59 ]. The observations confirmed that there was a significant correlation between the phenolic content and antioxidant activity. Another monocot, O. sanctum , showed that the inhibition of lipid peroxidation in vivo and in vitro increased proportionally with an increase in the concentration of the extract.
4.3. Antioxidants from Vegetables
Consumption of vegetables has been linked to a reduction in the risk of many diseases, such as cancer and cardiovascular disease, when studied in epidemiological studies [ 59 ]. Numerous studies have attempted to screen vegetables for antioxidant activity by using different oxidation systems. These vegetables include carrots, potatoes, sweet potatoes, red beets, cabbage, Brussels sprouts, broccoli, lettuce, spinach, onions, and tomatoes. In addition to the concise studies, which have used different methodologies to release bioactive compounds, it is becoming increasingly difficult to ignore advanced extraction methods, which have paved the way to extract bioactive compounds rapidly. Despite scientists’ successes in showing the activity of vegetables’ bioactive compounds, there is little known about the activity of the antioxidant components that have been isolated from these vegetables. Researchers have tended to focus on advanced methods to isolate and measure the activity of antioxidant compounds such as flavonoids, phenolic acids, tocopherols, carotenoids, and ascorbic acid [ 60 ].
4.4. Antioxidants from Fruits
Fruit consumption has also been linked to a reduction in the risk of many diseases [ 61 ]. Peaches ( Prunus persica L.) are an economically important fruit in many countries. Studies have shown that phenolic compounds found within various peach genotypes are a major source of potential antioxidants [ 60 ]. Interestingly, peaches have shown a great inhibition of low density lipoprotein (LDL) oxidation with a percentage of antioxidant activity of 56–87%. This antioxidant activity can be attributed to its essential compound content including hydroxycinnamic acids, chlorogenic, and neochlorogenic acids, but not to carotenoids such as b-carotene and b-cryptoxanthin. Moreover, low antioxidant activity was found in peach peel. In contrast, Plumb et al. pointed out that hydroxycinnamic acids do not contribute to the inhibition of lipid peroxidation of the liver using plums and peaches because hydroxycinnamic acids had weak ability to scavenge hydroxyl radicals [ 62 ].
Grape ( Vitis vinifera L.) is a fruit crop grown throughout the world. Grapes and its juices have been recently studied by [ 62 ]. Phenolic compounds were high in both fresh grapes and commercial grape juices. The percentage of inhibition LDL oxidation was about 22% to 60% for fresh grapes, while it was approximately 68% to 75% for commercial grape juices, when standardized at 10 mg gallic acid equivalents (GAE). According to [ 63 ], both grapes and its juices exhibited high oxygen radical absorbance capacity (ORAC), and the anthocyanin pigment malvidin-3,5-diglucoside was a major compound isolated in grapes. Anthocyanins with malvidin nucleus malvidin 3- O -(6- O -p-coumaroylglucosido)-5-glucoside and phenolics were common compounds isolated from wild grapes ( Vitis coignetiae ). Wangensteen et al. tested the activity of many bioactive compounds by releasing them from grape pomace, and demonstrated that bioactive compounds have the ability to significantly inhibit LDL oxidation in the human body [ 64 ]. Grape seeds are an amazing source of polyphenol compounds including monomerics such as catechin, epicatechin, and gallic acid, and polymerics such as procyanidins [ 65 ].
Both polyphenols and carotenoids are the major phenolic compounds of apples ( Malus domestica L.) including caffeic, quinic, and p-coumaric acids. These polyphenols can act as antioxidants. Flavanol monomers and oligomers, as well as quercetin, contribute to the beneficial health aspects of fruits and vegetables [ 65 ]. Apple pomace has mainly been used as a major source of polyphenols such as chlorogenic acid [ 66 , 67 ]. In addition phenolics like caffeic, p-coumaroyl quinic, arbutin, p-coumaric acids, and especially flavonol procyanidins have been mentioned as constituents of apple pomace [ 68 ]. The ability of procyanidins to work as oxygen radical scavengers, superoxides, and hydroxyl radicals was estimated. Despite the low content in total phenols in apples obtained by using acetone 70%, it has shown strong antioxidant activities towards oxidation of linoleic acid. In this case, the major bioactive compounds obtained were chlorogenic acid and phloretin glycosides; however, Vitamin C was a minor fraction in apple juice [ 69 ].
Antioxidant and antibacterial activities of various solvent (ethyl acetate, acetone, methanol, and water) extracts of Punica granatum peel were examined by applying the 2,2-diphenyl-1-picrylhydrazyl (DPPH) radical scavenging method. The results obtained showed a significantly higher decreasing power in the methanol extracts and a significantly higher antibacterial activity in the acetone extracts.
Soong and Barlow investigated the antioxidant activity and phenolic content of various fruit seeds [ 70 ]. Petroleum ether was used to get rid of the excess fat from the seeds and extraction has been carried out with methanol. The 2,2-azino-bis-3-ethylbenzthiazoline-6-sulfonic acid (ABTS), DPPH, and the ferric reducing ability of plasma (FRAP) methods were used to investigate the antioxidant activity. Abdille et al. examined the antioxidant activity of Dillenia indica fruit using different kinds of solvents using DPPH, phospho-molybdenum, and β carotene bleaching methods [ 71 ]. The methanol extracts showed the highest antioxidant activity, followed by the ethyl acetate and water extracts. Antioxidant activity of Syzygium cumini fruit in vitro has been investigated [ 71 ]. Antioxidant activity was measured by DPPH, superoxide, lipid peroxidation, and hydroxyl radical scavenging activity methods. The results brought to light a significant correlation between the concentration of the extract and the percentage of inhibition of free radicals. The antioxidant property of the fruit might be from the presence of antioxidant vitamins, anthocyanins, phenolics, and tannins. It has been reported that blackberry ( Rubus fruticosus L.) fruit extracts produced in varying climatic regions showed that antioxidant activity depended on the genotype, rather than the climate or season [ 10 ]. Juntachote and Berghofer measured the stability of the antioxidant activity of ethanolic extracts for Holy basil and galangal using DPPH, superoxide, β carotene bleaching, reducing power, and iron chelation methods [ 72 ]. They found higher antioxidant activity at neutral pH compared to an acidic pH. Holy basil and galangal extracts provided strong iron chelation activity, superoxide anion scavenging activity, and reducing power proportional to the concentration of the extracts. Liyana-Pathirana et al. investigated the antioxidant activity of cherry laurel fruit ( Laurocerasus officinalis Roem) and its concentrated juice (Pekmez) using in vitro methods such as superoxide, DPPH scavenging activity, and inhibition of LDL oxidation [ 73 ]. The results confirmed the presence of a significantly higher antioxidant activity in pekmez compared to the cherry laurel fruit. Employing in vitro methods such as DPPH and superoxide scavenging activity, Orhan et al. measured the antioxidant activity of Arnebia densiflora Ledeb and observed that polar extracts had a higher antioxidant activity compared to non-polar extracts [ 74 ]. Rathee et al. studied the antioxidant activity of Mammea longifolia buds extracted in both methanol and aqueous ethanol. The results found a significant antioxidant activity, and the activity of aqueous ethanol was higher than methanol. The antioxidant activity of leaf extracts of Annona species in vitro reveals that Annona muricata possessed a higher antioxidant activity compared to Annona squomosa [ 75 ].
4.5. Cooking Herbs as an Important Source of Antioxidants
The antioxidant activity of 32 herbs belonging to 21 different families has been screened [ 76 ]. The finding confirmed that there was a positive correlation between the total antioxidant activity and total phenolic content. Lu and Yeap Foo studied Salvia officinalis (L.) for its antioxidant activity and polyphenol content and reported that rosmarinic acid and various catechols were responsible for the radical scavenging activity and caffeic acid was responsible for the xanthine oxidase (EC 1.17.3.2) inhibition [ 77 ]. Zhao et al. investigated the antioxidant activity of Salvia miltiorrhiza and Panax notogensing [ 78 ]. The results showed that Salvia miltiorrhiza had a higher reducing power and scavenging activities against free radicals, including superoxide and hydroxyl radicals, although it showed weak hydrogen peroxide scavenging.
Furthermore, Javanmardi et al. tested the Iranian Ocimum sp. accessions to determine the antioxidant activities and total phenolic contents and demonstrated that the antioxidant activity increased in parallel with the total phenolic content [ 51 ].
Evaluation of the pomegranate peel extracts to discover its antioxidant and antimutagenic activities using different solvents such as ethyl acetate, acetone, methanol and water has been carried out [ 51 ]. Dried extracts were examined by using the Ames test and the phosphorus-molybdenum method to test both anti-mutagenic and antioxidant activities. The results showed the highest anti-mutagenic and the lowest antioxidant activity in the water extract.
Moreover, the phenolic content and antioxidant activity of parsley ( Petroselinum crispum ) and cilantro ( Coriandrum sativum ) have been tested [ 79 ]. The total phenolic content was observed to be different between parsley and cilantro leaves and stems, as well as methanol and water extracts. The methanol leaf extracts exhibited significant antioxidant activity towards both lipid- and water-soluble radicals. The works also investigated the antioxidant activity of aqueous plant extracts using in vitro methods such as DPPH scavenging activity and FRAP. The results revealed a strong correlation between total antioxidant activity and phenolic content and a weak correlation between cupric ion chelators and polyphenols. The antioxidant activity and lipid peroxidation inhibition of Satureja montana L. subsp. Kitaibelii extracts were tested using hydroxyl radical scavenging. The results obtained showed that there was a significant correlation with total phenolic content [ 9 ].
4.6. Antioxidant from Legumes
Antioxidant property of methanol extracts of Mucuna pruriens L. ( Fabaceae ) seed extracts has been investigated in vitro using the DPPH radical scavenging method. The results obtained showed a positive correlation between the antioxidant activity and the total phenolic compounds [ 80 ]. Siddhuraju and Manian studied horsegram ( Macrotyloma uniflorum Lam.) seeds to measure the antioxidant and free radical scavenging activity [ 81 ]. Acetone extracts had a higher activity of about 70% [ 81 ]. Samak et al. studied Wagatea sp. to measure its scavenging activities of superoxide and hydroxyl radicals and showed a high oxidation inhibition because it was rich in both phenolic and flavonoid contents. The authors also reported that bark and leaf extracts of Wagatea sp. exhibited high scavenging action against super radicals [ 82 ].
4.7. Antioxidants from Trees
Antioxidants from trees have been also measured. Phenolics from almond hulls ( Prunus amygdalus L.) and pine sawdust ( Pinus pinaster L.) have been extracted employing various methods in order to determine the gram fresh yield of polyphenol compounds and antioxidant activity [ 83 ]. The antioxidant activity was measured by the DPPH radical scavenging method. The results showed that ethanol was most appropriate either for phenolics or any bioactive compounds, while methanol was more selective for extracting polyphenolics. The antioxidant activity of juniper ( Juniperus communes ) fruit extracts has been investigated in vitro [ 84 ]. The results confirmed that both water and ethanol extract showed strong antioxidant activity. The concentration of 60 μg/mL of water and ethanol extracts exhibited 84% and 92% inhibition, respectively, on the peroxidation of linoleic acid. Ibrahim et al. studied the antioxidant activity of Cupressus sempervirens L., and set up goals to isolate quercetin, rutin, cupress flavone, caffeic acid, and para-coumaric acid. The results showed higher antioxidant activity related to quench DPPH and identified these active compounds successively [ 85 ].
Higher values of antioxidant activity have been obtained by using a methanolic solvent to extract the bioactive compounds from Anacardium occidentale , while other solvents like ethyl acetate gave lower values of antioxidant activity [ 85 ]. Kaur et al. studied the Chickrassy Chukrasia tabularis A. Juss leaves to confirm its ability to inhibit lipid peroxidation and showed that there was a large inhibition considering its high content of phenolic compounds [ 86 ]. Finally, Acacia nilotica L. antioxidant activity has been measured using ethyl acetate as a solvent to extract phenolic compounds [ 86 ]. The results exhibited the highest antioxidant activity when the concentration of extracts was relatively high.
4.8. Antioxidant from Shrubs
Many shrubs have been shown to contain antioxidant activity. Singh et al. tested several plants to measure the antioxidant activity from different extracts. The antioxidant activity was determined by using peroxide value, thiobarbituric acid, DPPH radical scavenging activity, and reducing power. The results showed that the antioxidant activity of Coriandrum sativum L. and Sarcolobus globosus L. exhibited high activity by using acetone solvent, and its activity was similar to synthetic antioxidants [ 87 ].
Eleven Algerian medicinal plants have been measured for phenolic compound content and antioxidant activity using the ABTS method. The tested plants showed antioxidant activity. Artemisia campestris L. had better antioxidant activity than caffeic acid and tocopherol. Moreover, HPLC analyses exhibited a good correlation between the antioxidant activity and hydroxycinnamic derivative content.
Evaluation of Vitex negundo Linn seed antioxidant activity using different methods such as superoxide, hydroxyl, and DPPH scavenging activity has been carried out [ 87 ]. The highest antioxidant activity was in both raw and dry heated seed extracts, while lower antioxidant activity was observed in the hydrothermally processed samples.
4.9. Characterization of Antioxidants from Other Eudicots
The nitric oxide and superoxide scavenging activity of green tea have been studied by Nakagawa and Yokozawa [ 88 ], who concluded that certain tannins had the ability to exhibit excellent antioxidant activity. Zin et al. estimated the antioxidant activity of the extracts from various parts of Mengkudu ( Morinda citrifolia L.), including the leaves, fruits, and roots, using different solvents such as methanol and ethyl acetate [ 89 ]. Ferric thiocyanate and thiobarbituric acid were used as models to observe and evaluate the antioxidant activity. The results exhibited a higher antioxidant activity in the methanol extract of Mengkudu root, although it was not significantly different from tocopherol and BHT extracts. The methanol extracts of the fruits and leaves showed unassuming activity. According to these scientists, the antioxidant activity in the roots resulted from polar and non-polar compounds, but the antioxidant activity in leaves and fruits was only due to non-polar compounds.
Increase of the antioxidant activity of fennel ( Foeniculum vulgare ) seed extracts in vitro has been shown to be proportional to the increase in the concentration of extract [ 89 ]. Nine other extracts of Bolivian plants have been measured for radical scavenging and antioxidant activity using the DPPH and β carotene bleaching methods [ 90 ]. It was found that the ethyl acetate fractions had higher radical scavenging and antioxidant activity compared to the other extracts. It has been reported that the bioactive compounds of Rhodiola rosea extracted in methanol showed a significant yield of phenolics, about (153 ± 2 mg/g) [ 91 ]. Wangensteen et al. investigated the antioxidant activity of Ss globosus using DPPH scavenging and inhibition of lipoxygenase [ 64 ]. Coriander had a high capacity to inhibit oxidation. There was also a positive correlation between total phenolics and antioxidant activity. Moreover, it was observed that the leaves of the coriander had higher antioxidant activity than the seeds [ 91 ].
Antioxidant activity of Phyllanthus niruri was estimated using methanol and water as a solvent. The extracts of leaves and fruits exhibited high antioxidant activity by using the inhibition of lipid peroxidation and DPPH scavenging [ 64 ]. The results also noticed a higher superoxide scavenging activity in the aqueous extract compared to the methanol extract. Moreover, the antioxidant and free radical scavenging activity of Phyllantus species from India in an aqueous extract has been also evaluated [ 92 ]. The antioxidant activity was estimated using DPPH, β carotene, superoxide, nitric oxide scavenging, and reducing power methods. The extract of Coleus aromaticus exhibited a moderate inhibition on DPPH and nitric oxide scavenging activity.
Panax exhibited strong iron chelating and weak superoxide scavenging. Ajila et al. carried out bioactive compounds and antioxidant activity of mango peel extract [ 93 ]. The results showed a higher concentration of anthocyanins and carotenoids in the ripe peel compared to the raw peel, while the raw peel exhibited higher polyphenol content. The range of IC50 values of lipid peroxidation and DPPH were 1.39–5.24 μg of gallic acid equivalent. Chen and Yen investigated the antioxidant activity and free radical scavenging capacity of dried guava leaves and fruit [ 94 ]. The results confirmed that guava leaf and guava tea extracts had the ability to inhibit oxidation by 94–96% at a concentration of 100 μg/mL. Fruit extracts exhibited less activity compared to leaf extracts, while the scavenging effect increased with an increase in the concentration. Also, there was a correlation between antioxidant activity and phenolic compounds. Dastmalchi et al. investigated the chemical composition and antioxidant activity of water-soluble Moldavian balm ( Dracocephalum moldavica ) in vitro by using DPPH, ABTS, and superoxide activity [ 95 ]. The finding confirmed that polar compounds such as caffeic acid and rosmaric acid were responsible for the antioxidant activity observed.
Mulberry leaves were investigated to determine the antioxidant activity using different solvents [ 95 ]. The procedure used DPPH and inhibition of lipid peroxidation methods to evaluate its activity. The results showed that the methanolic extract exhibited the highest yield of total phenolics, and it was the most essential antioxidant in all the methods used. The antioxidant activity of kale ( Brassica obraceae L.) has been screened after removing a fat fraction from the samples [ 96 ]. The extraction process used methanol to investigate its antioxidant activity while using DPPH scavenging activity as tested method. The works successfully isolated nine phenolic acids using HPLC and MS, and confirmed that the total phenolic content was correlated with DPPH scavenging activity.
In another study, ethanol has been used to estimate the antioxidant activity of sun-dried cashew nuts ( Anacardium occidentale L.) skin [ 97 ]. First, bioactive compounds were extracted with a protocol including lipid peroxidation, ABTS, and DPPH methods to measure the capability to inhibit oxidation. The results found that epicatechin was the major polyphenol in the extract, which was responsible for antioxidant activity.
Kaviarasan et al. measured the antioxidant and antiradical activity of fenugreek ( Trigonella foenum ssp. graecum ) seeds in vitro; the results showed that there was a positive relationship between the antiradical activity and phenolic compound content in the extract [ 98 ]. Hexane and methanol were used to extract the bioactive compounds and measured the antioxidant activity of Pueraria tuberosa by using ABTS, lipid peroxidation, and superoxide and hydroxyl scavenging activity. An independent study has shown an inhibition of the lipid peroxidation [ 99 ].
The rhizome of the lotus ( Nelumbo nucifera Gaertn.) has been measured for its antioxidant activity in various solvent extracts using β Carotene bleaching and DPPH methods [ 99 ]. Methanol extraction had a higher DPPH scavenging activity than acetone. Helichrysum pedunculatum has been tested to determine the antioxidant activity, and total phenolic and flavonoid content [ 100 ]. The results demonstrated that whenever the amount of phenolic content and flavonoid content was increased, higher antioxidant activity was obtained. Meot-Duros and Magn screened the leaves of Crithmum maritmum to show if there was any correlation between the antioxidant activity and phenolic content and found a significant correlation between antioxidant activity and phenolic content when methanol was used as the solvent [ 101 ].
Another dicot, Tricholepis glaberrima L. ( Asteraceae ), has been investigated for antioxidant activity using different kinds of extracts [ 101 ]. Higher antioxidant activity was found by methanol, and a lower antioxidant activity in both chloroform and aqueous extracts. Sakat et al. investigated Oxalis corniculata L. in order to measure the antioxidant and anti-inflammatory activity employing methanol as a solvent. The IC 50 values of DPPH and nitric acid were about 93 and 73.07 μg/mL, respectively [ 102 ].
Jain et al. studied Tabernaemontana divaricata L. to determine the phytochemical and free radical scavenging activities in vitro. The results indicated that the antioxidant activity was the same in both ethanol and water extracts, but less in petroleum ether [ 103 ].
It has been reported that Ascleipiadaceae and Periplocoideae presented high antioxidant activity, with the presence of a strong correlation between antioxidant activity and phenolic content [ 103 ]. Laitonjam and Kongbrailatpam studied the chemical composition and antioxidant activities of Smilax lanceafolia by isolating the flavonol glycoside and steroidal saponin, which showed high antioxidant activity [ 104 ]. Spinach ( Spinacea olerace L.) is among the most popular vegetables in the world. It was domesticated and first cultivated in West Asia. According to analytical chemistry, spinach is a source of violaxanthin and neoxanthin antioxidants that cannot be commercially produced [ 105 ]. Although they may be present, pigments such as carotenoids can be masked by chlorophyll in greenish vegetables such as spinach [ 106 ]. B-carotene, lutein, violaxanthin, and neoxanthin are the major carotenoids in raw spinach [ 107 ]. Pumpkins belong to the family Cucurbitaceae . This family is classified depending on the texture and shape of stems, such as in Cucurbita pepo , Cucurbita moschata , Cucurbita maxima , and Cucurbita mixta . Nowadays, the market offers a wide variety of vegetables, with pumpkin being one of them because of its many applications for nutrition or decoration [ 108 ].
5. Plants Vitamins and Phenolic Compounds as Antioxidants
5.1. phenolic compounds, 5.1.1. phenols and phenolic acid.
Phenolic acids contain carboxylic acid in the chemical composition. Hydroxycinnamic and hydroxybenzoic acids are both main pillars of phenolic acids, according to Figure 1 A. Moreover, scientists have noted that p-coumaric, caffeic, ferulic, and sinapic acids are main components of the hydroxycinnamic acids ( Figure 1 A).

Chemical structure of phenolic acid ( A ), flavonoids ( B ), anthocyanins ( C ), and tannins ( D ).
5.1.2. Flavonoids
Flavonoids have a low molecular weight ( Figure 1 B). Flavane is an example of a flavonoid. Flavane contains two benzene rings ( Figure 1 A,B) within its chemical composition. These two rings connect to each other through a pyrane ring ( Figure 1 C). Flavones, isoflavones, flavonoids, flavonols, flavanones, anthocyanins, and proanthocyanidins are part of flavonoids according to the flavonoid classification ( Figure 1 B).
5.1.3. Anthocyanins
Anthocyanidins are a simple example of anthocyanins. Anthocyanidins consist of an aromatic ring that is linked to a heterocyclic ring ( Figure 1 C). Moreover, the heterocyclic ring is connected to the third aromatic ring through a carbon bond [ 109 ]. Scientists have noted that anthocyanins are often found in a glycoside form. Moreover, many kinds of anthocyanins are found in nature, making these kinds of phenolic compounds very complex. Scientists have noted that anthocyanins in different kinds of fruit are considered an essential compound that can enrich and increase antioxidant activity ( Figure 1 C).
5.1.4. Tannins
Tannins are natural products present in several plant families, and have large amounts of phenolic rings in the structure. Tannins are classified into two groups: hydrolyzable and condensed. Condensed tannins contain flavonoids units with several degrees of condensation. Hydrolyzable tannins are considered a mixture of simple phenols with ester linkages in its structure. There are many factors such as alkaline compounds, mineral acids, and enzymes that have the ability to hydrolyze tannins ( Figure 1 D) [ 110 ].
5.2. Vitamins Role in Cancer Prevention
Cancer has been increasing throughout the world. It is the main cause of mortality from year to year. There were 10.4 million new cancer cases registered in 2015, and scientists predict that the number of cancer cases per year will double by 2030 [ 111 ]. Recently, many studies have shown rigorous evidence that hydroxyl radicals (OH•) and the superoxide anion ( O 2 − •) are involved in the development of cancer because they are biological reactive oxygen species. Compounds with high reactive oxygen species reduction activity are likely able to prevent cancer’s occurrence [ 112 ]. As shown previously, fruits and vegetables are the primary source of natural antioxidants, consisting of different kinds of antioxidant compounds such as Vitamin C, Vitamin E, carotenoids, lutein, and lycopene. Some researchers have confirmed that phenolic compounds and polyphenols are secondary plant metabolites, which are considered the best scavengers to prevent the production of free radicals. The United States has an amazing diversity of plant species. Some of them have been used for traditional medicines for a long period of time because of their various desirable activities. Kiwi and pomegranate plants extracts were screened to show the cytotoxic effects on two tumor cell lines ( L20B and RD ). The results have shown that the means of both L20B and RD cultures were significantly different ( p < 0.05), and kiwi and pomegranate plant extracts exhibited a strong ability to inhibit the growth rate of L20B and RD cell lines. At concentrations of 1000 μg/mL, both extracts showed a high ability to decrease the number of L20B and RD cells when compared with the control [ 113 ].
The mixtures of the plant natural products have been screened in order to study their effect on human leukemia cells [ 114 ]. The finding confirmed that mixtures of natural products were a good source for human leukemia cell inhibition. Nassr-allah et al. investigated the chemical diversity of natural products from plants in order to test their ability to work as anticancer and antioxidant agents [ 115 ]. DPPH assay was used to measure the antioxidant activity for plant extraction while using in vivo and vitro methods in order to measure the anticancer activity. The results confirmed that some natural products from Egyptian flora have the potential for use as therapeutics for diseases such as cancer [ 116 ].
The effectiveness of an aqueous extract from willow leaves ( Salix safsaf , Salicaceae ) against human carcinoma cells has been tested in vivo and in vitro [ 115 ]. The findings mentioned that the metabolites for the willow extract could inhibit tumors, thereby enhancing apoptosis and causing DNA damage. The anticancer activity of different extracts from the leaves of the drumstick tree ( Moringa oleifera ) was screened in order to test against leukemia and hepatocarcinoma cells in vitro. Primary cells harvested from 10 patients with acute lymphoblastic leukemia (ALL) and 15 with acute myeloid leukemia (AML) were significantly killed by hot water and ethanol extracts. Thus, Moringa oleifera may have the potential for use as a natural treatment for diseases such as cancer [ 117 ]. Altemimi reported that the phenolic extracts from the olive leaf extract could be used as a source of potential antioxidant and antimicrobial agents [ 118 ].
6. Plants as an Antimicrobial Source
The antibacterial activity of Punica granatum extracts has been investigated by using various solvents [ 119 ]. The water extract had the ability to inhibit Bacillus subtilis and Staphylococcus aureus , but the organic solvents have the ability to inhibit the growth of all the organisms tested. Shariff et al. estimated the antibacterial activity of Rauvolfia tetraphylla and Physalis minima leaves. The chloroform extract was a more powerful inhibitor of pathogenic bacteria [ 120 ].
Indian medicinal plants have been shown to have antimicrobial activity [ 120 ]. About 77 extracts belonging to these plants have been tested for their antimicrobial ability against eight species of bacteria and four species of pathogenic fungi. The findings showed that water extracts of Lantana camara L., Saraca asoca L., Acacia nilotica L., and Justicia zeylanica L. caused the highest growth inhibition of all tested bacteria. The antimicrobial activity was the highest, ranging between 9.375 and 37.5 μg/mL and 75.0 to 300.0 μg/mL against both bacterial and fungal pathogens.
Devi et al. investigated Achyranthes bidentata Blume to determine its phytochemical content and antibacterial activity [ 121 ]. The antibacterial ability of the ethanol extract effectively inhibited Bacillus subtilis , Salmonella typhi , and Klebsiella pneumoniae , but was less effective against Pseudomonas species and Staphylococcus aureus [ 122 ]. Ethanolic extracts of Gymnema montanum L. have been studied to measure its antimicrobial properties against Salmonella typhi , Pseudomonas aeruginosa , and Candida albicans [ 121 ]. The results indicated the highest presence of antimicrobial properties in the leaf extract of G. montanum, correlating to its phenolic compound content. The antimicrobial activity of Piper ribesoides L. from methanolic root extract against Staphylococcus aureus has been reported [ 123 ]. Interestingly, a small amount of 3.125 mg/mL was enough to inhibit harmful bacteria. Leaf extracts of Caesalpinnia pulcherrimma (L.) showed higher antioxidant activity in water and ethanol extracts and lower antioxidant activity in petroleum ether extracts [ 124 ]. Torilis japonica L. fruit has been observed to reduce the amount of spores, and the concentration of the vegetative cell was lower than the detection level. Ghosh et al. studied Stevia rebaudiana Bertoni to measure its antimicrobial properties against 10 pathogens [ 125 ]. The findings confirmed that Staphylococcus aureus was more susceptible than others [ 24 ]. Mahesh and Satish screened some important medicinal plants to show the antibacterial activity on human pathogenic bacteria [ 126 ]. Water and methanol were used as solvents to extract the phenolic compounds. The finding confirmed that the methanol extract had a higher antimicrobial activity than the aqueous extract [ 125 ].
Moreover, leaf extracts of Acacia nilotica L., Sida cordifolia L., Tinospora cordifolia L., Withania somnifera L., and Ziziphus mauritiana L. have been studied to determine the antibacterial activity against Bacillus subtlis , Escherichia coli , Staphylococus aureus , and Pseudomonas fluorescens , as well as studying the antifungal activity against Aspergillus flavus , Dreschlera turcica , and Fusarium verticilloides [ 126 ]. The highest antibacterial activity was noticed in Acacia nilotica and Sida cordifoliain leaves, and the highest antifungal activity was noticed in Acacia nilotica bark. Water and methanol extracts of Samanea saman (Jacq.) exhibited a significant effect against Xanthomonas spp. and human pathogenic bacteria. Pseudarthria viscida root has been studied to measure its antimicrobial activity using ethanol as a solvent. The results showed high antimicrobial activity when compared to standard drugs like ciprofloxacin and griseofulvin.
Ehsan et al. reported a high antimicrobial activity against Staphylococcus aureus using methanol and ethanol extracts for Hopea pariviflora Beddome [ 127 ]. Ethanolic extracts of Bryonopsis laciniosa have been investigated for their antimicrobial activity against different Gram-positive and Gram-negative bacteria. The growth of Staphylococcus aureus , Micrococus luteus , and Bacillus cereus was inhibited, as shown by a decrease in the growth zone.
Plumbago zeylanica L. has been screened to measure the antibacterial activity in chloroform extracts to show antimicrobial activity against Escherichia coli , S. typhi , and Staphylococcus aureus [ 127 ]. However, Bacillus subtilis and Klebsiella were resistant. Khond et al. studied 55 medicinal plants to measure the antimicrobial activity [ 128 ]. The higher antibacterial activities were in the extracts of Madhuca longifolia L., Parkia biglandulosa L., and Pterospermum acerifolium L. compared to the other plants screened. Pavithra et al. screened Evolvulus nummularius L. for its antibacterial activity, finding that Escherichia coli and B. subtilis were the most inhibited by an ethanolic extract [ 129 ].
Hygrophila spinosa Andres leaves showed significant antibacterial activity when collected between September to October, with less activity seen during other months [ 129 ]. Artemisia pallens L. has been studied for its antimicrobial activity against seven species of bacteria [ 130 ]. The results found that Bacillus cereus was more sensitive to A. pallens extracts. Also, a methanolic extract exhibited higher antibacterial activity than the other solvents used. Akroum indicated the antimicrobial activity of some Algerian plants [ 131 ]. The results expressed higher antibacterial activity in methanolic extracts of Linum capitatum , Camellia sinensis , Allium schoenoprasum , Vicia faba , Citrus paradise , Lippia citriodora , Vaccinium macrocarpon , and Punica granatum . Bajpai et al. screened the antibacterial activity of Pongamia pinnata leaves by using methanol and ethyl acetate extracts to confirm its ability against certain pathogenic bacteria [ 132 ]. The results exhibited significant inhibition compared to streptomycin. It has been demonstrated that Memecylon edule has higher antibacterial activity in chloroform extracts compared to other extracts [ 132 ]. Gram-negative bacteria were more susceptible to the crude extracts compared to Gram-positive bacteria. Bansal et al. studied plants found in arid zones in order to determine the antibacterial efficiency [ 133 ]. An ethanolic extract of Tinospora cordifolia L. inhibited Bacillus cereus and Staphylococcus aureus . Kumar et al. reported Andrographis serpyllifolia L. to have significant antimicrobial activity against tested organisms in methanol extracts of both aerial parts and root [ 134 ].
Memecylon malabaricum , Cochlospermum religiosum, and Andrographis serpyllifolia have been rested for their possible antimicrobial activity [ 135 , 136 ]. Moderate activity against both Gram-positive and Gram-negative bacteria was observed. The antimicrobial activity of an ethanolic extract of Anethum graveolens was better than the aqueous extract. Khanahmadi et al. [ 137 ] found a higher antibacterial activity against Gram-positive bacteria compared to Gram-negative bacteria when an ethanolic extract of Smyrnium cordifolium Boiss was used [ 136 , 137 ]. Koperuncholan et al. studied some medicinal plants of the south eastern slopes of the Western Ghats [ 138 ]. Gram-positive bacteria were more sensitive than Gram-negative bacteria to the plant extracts. Niranjan et al. screened Schrebera swietenioides Roxb to measure the effectiveness against human pathogenic bacteria [ 139 ]. Water and methanol extracts were most effective to prohibit growth of all the harmful bacteria tested.
Different studies have isolated tannins and saponins from some Indian medicinal plants, testing the antibacterial activity against Klebsiella pneumoniae [ 139 , 140 ]. Ethanol extracts of Tinospora cordifolia strongly inhibited Bacillus cereus and Staphylococcus aureus . Also, significant antibacterial activity from ethanolic extracts of Coleus aromaticus L. has been found. The most effective range of inhibition was at concentrations of 25–39 μg/mL. Vinothkumar et al. evaluated a Andrographis paniculata L. leaf extract’s ability to inhibit the growth of Gram-positive and Gram-negative bacteria. The results found that aqueous extracts inhibited harmful microbes [ 134 ].
A positive effect of pumpkin has been observed by investigating its antimicrobial activity against Staphylococcus aureus , Bacillus subtillus , Escherichia coli, and Pseudomonas . aeruginosa . Three different solvents were used to prepare the extracts: water, chloroform, and alcohol. The results showed that the alcohol extract was more powerful than both water and chloroform extracts. Staphylococcus aureus was sensitive to all extracts. Recently, the novel antimicrobial activity of ultrasonicated spinach leaf extracts using random amplification of polymorphic DNA (RAPD) markers and electron microscopy against both Gram-positive and Gram-negative bacteria has been revealed [ 134 ]. RAPD is an emerging technique used for diagnostic mutation detection within a genome. The range of the minimum inhibitory concentrations (MICs) of the extracted leaf spinach antimicrobial substances against Escherichia coli and Staphylococcus aureus was observed between 60 and 100 mg/mL. The optimal extraction conditions were at 45 °C, ultrasound power of 44%, and an extraction time of 23 minutes. The study showed that the treated bacterial cells appeared to be damaged by a reduction in cell number. In fact, it was inferred that spinach leaf extracts exert bactericidal activity by inducing mutations in DNA and causing cell wall disruptions.
7. Conclusions
In summary, plant extracts showed strong antioxidant capacity both in vitro and in vivo, and the extracts can be considered a good source of natural antioxidants and antimicrobials. Polyphenol extraction from plants using fast and appropriate techniques is a low-cost method due to the reduction in the amount of solvent used, in addition to avoiding the need for longer extraction times compared to the conventional extraction method. Moreover, natural bioactive compounds have been found to interfere with and prevent all kinds of cancer. Flavonoids have been shown to work as anti-tumor (benign, melanoma) agents involving a free radicals quenching mechanism (i.e., OH, ROO). In fact, many studies have shown that flavonoids play significant multiple roles including mutagenic, cell damage, and carcinogenic, due to their acceleration of different aging factors. In addition to antioxidant activity, the inhibition of cancer development by phenolic compounds relies on a number of basic cellular mechanisms. More comprehensive studies related to these compounds will enhance pharmaceutical exploration in the field of carcinogenic disease prevention.
Acknowledgments
The authors would like to thank the Higher Committee for Education Development in Iraq (HCED) for the financial support to achieve this work and Stuart Alan Walters of the Department of Plant, Soil and Agricultural Systems, College of Agricultural Sciences, Southern Illinois University.
Supplementary Materials
The following are available online at www.mdpi.com/2223-7747/6/4/42/s1 .
Author Contributions
Ammar Altemimi drafted the manuscript; Naoufal Lakhssassi and Azam Baharlouei edited the manuscript and made the corresponding figures and tables; Dennis G. Watson and David A. Lightfoot edited and commented on the manuscript. All authors have read and approved the final manuscript.
Conflicts of Interest
The authors declare no conflicts of interest.

Want to create or adapt books like this? Learn more about how Pressbooks supports open publishing practices.
5 Research Project: Pigments in Plant Leaves
To determine the composition of pigments in different types of plant leaves.
Expected Learning Outcomes
- Extract, separate, and characterize compounds from a mixture.
- Describe the purpose of analytical separations.
- Conduct thin-layer chromatography (TLC), column chromatography and high performance liquid chromatography (HPLC).
Reading Assignment
The focus of this experiment is on liquid phase extractions/separations. While separations are mentioned briefly in Tro, Chemistry – Structures and Properties , 2nd Ed, Ch. 1.2, the following readings from Nichols, L., Organic Chemistry Lab Techniques will help you understand this experiment:
- Chromatography: Ch. 2.2 , 2.3(e) , 2.4(b)
- Extraction: Ch. 4.2 , 4.3
In addition, we will introduce HPLC (high performance liquid chromatography. Please read Barkovitch, M., High Performance Liquid Chromatography and watch the following video produced by the Royal Society of Chemistry:
About This Project
So far, most of the experiments we have done have definite, well known answers, and we’ve been doing experiments that either (a) confirm what you’ve learnt or (b) illustrate concepts from the lecture course. We will continue to do those experiments, not least because the combination will support your learning and help you develop laboratory skills.
However, in real life, we often need to study problems with no well-defined solution or problem. We are dealing with unknown problems.
As you may know, there are a number of pigments in plant leaves (that make it the color they are) which are critical to photosynthesis. [1] However, there is a mixture of them, and as leaves have different colors, how would the pigments vary – what pigments are there, and how much? We will do this using two forms of column chromatography as explained below.
Chemical Principles
Most of the substances we find in nature (wood, air, petroleum, etc.) are not pure but are instead more or less complex mixtures. Some of these mixtures require little or no modification before being put to practical use, but many of them must be at least partially separated into their components before they can be used to the best advantage.
- Crude oil is a mix of different hydrocarbons (compounds formed from carbon and hydrogen) that is separated into different components as shown below.
- Hard water is “softened” by separating out dissolved calcium carbonate (limescale) from water.
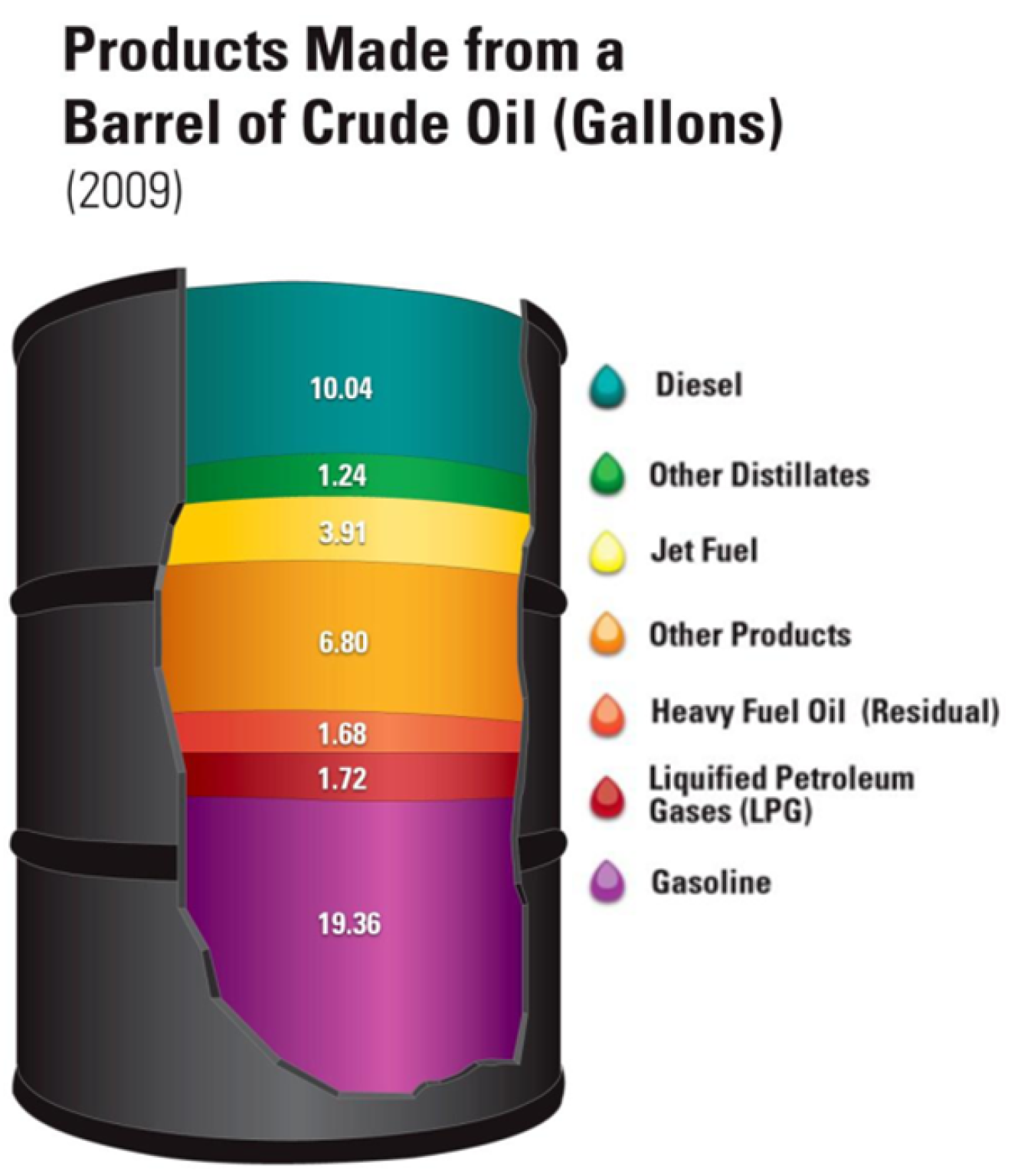
There are a number of chemical and physical processes used to separate mixtures but two of the most important for scientists today are the closely related techniques of extraction and chromatography.
Separation Method 1: Extraction
Extraction is the process of separating different substances by their differing solubilities in the same solvent (or solvent mixture).
When coffee is made using either a percolator or a drip-type coffee-maker, the hot water acts as a solvent to dissolve caffeine, tannic acid, and a large number of other chemical compounds, leaving the coffee grounds undissolved. This process is an extraction because of the differing solubilities of different substances: the caffeine and tannic acid are much more soluble in hot water than other components of the coffee grounds are (cellulose, for example).
Coffee-making illustrates the main requirements for a successful extraction:
- The mixture to be separated must be thoroughly mixed with the solvent (for example, by the “percolating” action).
- Other components must not dissolve in the solvent.
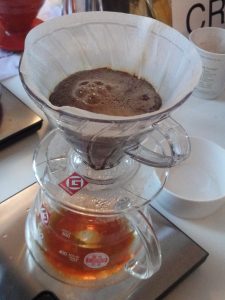
For a successful extraction we must find a solvent (or solvent mixture) that does a good job of dissolving the components we want but leaves undissolved components we don’t want. Since the solubility of most solids and liquids goes up with increasing temperature we can sometimes get the best results by using a hot solvent for the extraction. This is true for instance for most of the substances we want in our coffee, so we always start with very hot water (even if we are planning to end up with iced coffee). Other ways include using different solvents.
Separation Method 2: Chromatography
Chromatography is a general technique that has several points in common with extraction; for example, differing solubilities of different substances play a part in both techniques. Chromatography differs from extraction in that this always involves the movement of one phase (the “mobile phase”) past a non-moving phase (the “stationary phase”) to bring about the separation. Conditions are set up so that different components of the mixture are carried along with the mobile phase at different speeds as they pass through the stationary phase.
Different inks can be separated into different compounds/pigments using various forms of chromatography.

The big idea here is that there are two substances in which a substance can be dissolved:
- The stationary phase is a substance that is fixed along the entire structure where chromatography is conducted.
- The mobile phase which is typically a liquid or gas, and can move through the stationary phase.
Depending on the nature of the two phases and the substance concerned, there are varying ratios of solubility between the mobile phase and the stationary phase for different compounds. When a substance is more soluble in the mobile phase, it would tend to move along with the solvent front (the point where pure solvent has flowed to). Conversely, when a substance is more soluble in the stationary phase, then it would tend to stay on the stationary phase and not move.
For the inks on the TLC plate above, the extent to which the sample is soluble in the ethanol:water mixture relative to the silica (stationary phase, surface on the TLC plate) determines how far it migrates. Relatively speaking, the yellow ink is more soluble in ethanol:water (relative to silica) compared to the black ink.
While TLC is useful for quickly analyzing a sample and determining what compounds are present in that sample, it is very difficult to remove compounds from a TLC plate, thus it is not generally used to purify compounds. If you want to be able to collect the components of a mixture after separating them, column chromatography is more useful. In this technique, you place the stationary phase (for this experiment, silica gel) in a column (which is constructed using a glass, disposable Pasteur pipet). The sample is then loaded, and mobile phase is loaded to drive the sample until it elutes out of the bottom of the column. Each component is therefore isolated from each other and can be studied using absorption spectroscopy. This is the main method used to purify, among other things, proteins for biochemical experiments.

High Performance Liquid Chromatography (HPLC)
In this course, we have previously described how chromatography can be used to separate different components in a mixture. However, better separations can be made with columns created using specialized micrometer-sized particles (often silica). As the size of the particles decreases, the separation of the column improves.
However, with such fine particles the column would not function under room pressure. As such, high performance liquid chromatography (HPLC) instruments operate by forcing the solvent through the column under high pressure. In each case, the difference in affinity of solutes between the mobile and stationary phases is exploited to separate the substances.
While different column resins exploit different types of separation, one of the most common approaches to separation is by polarity of the solute. One of the most prevalent resins is that for reverse-phase HPLC (RP-HPLC), where a non-polar stationary phase is used in conjunction with a polar mobile phase. The most common resins for reverse phase HPLC are the octyl (C8) and the octadecyl (C18) columns.
The sample will then be detected by some means (often, UV-vis absorption) and a chromatogram is formed. Such a chromatogram is a plot of the signal as a function of time elapsed since the sample is injected.

Absorption Spectrum
Depending on the chemical structure of the molecule, different molecules can absorb light of different wavelengths. The color of the compound is the complementary color of the color of light absorbed – as seen in the color wheel, where the color observed is on the opposite side of the wheel:
In turn, each color of light corresponds to a different frequency/wavelength of light. The absorbance of light at different wavelengths can be plotted in an absorption spectrum , which can be used to help characterize a compound:
- This is a multi-week experiment. Your notebook will be graded when you finish the HPLC part of the experiment.
- It will be conducted in pairs.
- Before conducting this experiment, please review the directions on vacuum filtration and using the SpectroVis Plus in Using Laboratory Equipment .
- As this is a research project and particularly since it’s the first time we’ve tried this, the procedure is prone to revisions and change.
Week 1: Extraction and Thin-Layer Chromatography
This week, you will
- extract the pigments using organic solvents and hot water.
- analyze the extracted pigments using TLC.
Required Special Materials and Chemicals
- Mortar and pestle
- sand (a pinch)
- Sodium sulfate (15 g)
- saturated sodium chloride solution (2 mL – use from provided bottle)
- acetone (15 mL)
- hexane (15 mL)
- TLC plates (2)
- 110 mm diameter filter paper (2)
- forceps (1)
- Observe and record the appearance and species of the leaves. Take a photograph of the leaves.
- Weigh out 1.2 g of fresh leaves. Record the species and mass to the nearest 0.001 g, as well as a description of the leaves.
- Tear or cut the leaves into small pieces and place them in a mortar with a pinch of sand (to assist with grinding up the leaves). Add 6 mL of acetone and 6 mL of hexane and grind the mixture for 3–5 minutes in the fume hood. If little liquid is left in the mortar (<3 mL), add another 3-6 mL of each solvent and grind for another minute. You’re looking for at least 3 mL of a dark green liquid in your mortar.
- Filter the green solution using gravity filtration , into a clean 50 mL graduated cylinder then transfer it to a clean test tube. Note: do NOT filter directly into the test tube as it is likely that the test tube will break if you do.
- Add 2 mL of saturated aqueous sodium chloride solution to the test tube.
- Use a pipette and pipette the mixture up and down until it is well mixed.
- Place the test tube in the test tube rack and allow the mixture to separate.
Create a filtering pipet using a short (5″) disposable Pasteur pipet and cotton plug by tearing off a VERY SMALL (as small as possible) piece of a cotton ball, putting it into the top of the 5″ (short) Pasteur pipet and gently pushing it to the bottom of the pipet with a 2nd, longer (9″) pipet (the point of the cotton plug is just to prevent the sodium sulfate from falling out of the pipet, so don’t use too much cotton and don’t pack the cotton down too tightly). Fill the filtering pipet half-way with solid sodium sulfate using a weigh paper funnel. The final traces of water are removed by treating the organic solution with sodium sulfate as a drying agent.
- Filter the green organic layer through the filtering pipet into a screw cap vial by using a transfer pipet to move the green top layer from the test tube into the top of the filtering pipet and letting the liquid flow through the sodium sulfate and into the screw cap vial. Avoid adding any of the aqueous layer to the filtering pipet.
- Spot and run two TLC plates with your filtered green organic solution (see below for details).
- Loosely cap and label (initials, date, leaf pigment in hexane/acetone) the vial and store the vial in a small beaker (so it can’t tip over) in your drawer for next week. Be sure to NOT have the cap screwed on tightly, otherwise the solvent won’t fully evaporate. The solvent will evaporate away during this time leaving the pigment as residue. We will redissolve this residue next week.
Thin Layer Chromatography
Create the developing chambers:
- Place a 110 mm diameter filter paper into a 400 mL beaker such that it is pressed up against the side of the beaker and touching the bottom of the beaker (you will need to fold the filter paper over a bit to create a flat bottom in order to get it to fit into the beaker). You will need 2 beakers, 1 for hexane and 1 for acetone (I recommend labeling each beaker with tape so you don’t forget which beaker contains which solvent).
- Create a lid for each beaker out of aluminum foil by covering the top of the beaker with a square of aluminum foil and shaping it to the top of the beaker to seal it as tightly as possible.
- If you draw up and expel the eluent in your transfer pipet several times before transferring it to the beaker, the solvent won’t drip out of the pipet as much.
- Always hold pipets up and down (perpendicular to the floor), never sideways (parallel to the floor) as holding it sideways will cause the liquid to spill out.
- At this point, there should be a layer of solvent in that bottom of the beaker that’s ~0.5 cm thick. If it is significantly less than this, add a little more solvent.
Spot your TLC plates with your samples:
- Never touch the side of the TLC plate that has the silica gel with gloves. It can damage the silica gel and make the TLC plate unusable. Only touch the back (the plastic side) or the sides of the TLC plate.
- Never bend a TLC plate as this will cause the silica gel to flake off and make the TLC plate unusable.
- Using a NON-MECHANICAL PENCIL (the ink in pens will dissolve in organic solvents, and mechanical pencils will scratch off the silica gel), label the top of each of your TLC plates with your initials and the date.
- Label the bottom of your TLC plate with the eluent you will use to develop that plate (meaning one TLC plate should be labeled as hexane and the other one as acetone).
- Make 2 small horizontal lines 1 cm from the bottom of the TLC plate on each edge of the TLC plate (see fig. 1).
- Make 3 small (~1 mm) vertical tic marks 1 cm from the bottom of the TLC plate and ~0.5 cm away from the sides of the TLC plate and each other and label these tic marks “1”, “2”, and “3”.
- Don’t put your finger over the top opening of the capillary tube, doing so will prevent the liquid from going in and out of the tube.
- You can usually make 2-3 spots from a single dip into your organic solution, but if you’re concerned you won’t have enough solution left in your capillary tube to make your next spot (keep an eye on where the solvent level is in your capillary tube before and after making each spot), just dip the capillary tube back into your organic layer.
- After the organic solvent has evaporated from the spots on your TLC plate (when the spot is no longer as dark; this should only take a few seconds), create a 2nd spot of the same size on top of the spots in the “2” and “3” lane. This is known as double-spotting and is done if you’re concerned that adding a single spot won’t add enough of the compounds of desire to see after running.
- Add a 3rd spot to the “3” lane after the organic solvent has evaporated again. This means that you should now have 3 lanes, each with a single dot over the tic mark . The “1” lane is single-spotted, the “2” lane is double-spotted, and the “3” lane is triple-spotted. This way it is more likely that one of the lanes will have the right amount of the mixture to be able to see each compound as a distinct spot.
- Repeat this process of creating a single, double, and triple spot on the 2nd TLC plate.
Run your TLC plate:
- Place your prepared TLC plate into the appropriate developing chamber such that the bottom of the TLC plate is in the solvent and the top of the TLC plate is resting against the side of the chamber at a ~45-degree angle and immediately replace the aluminum foil lid on top of the beaker (seal as tightly as possible).
- Make sure that the TLC plate is oriented such that you can easily watch the solvent travel up the plate (places with solvent will be darker).
- Make sure the solvent level is well below where you spotted your compounds, otherwise they can dissolve into the solvent. If this happens, you will need to make a new eluent chamber and a new TLC plate.
- I’ve found that 10 minutes usually comes first.
- During these 10 minutes, you should be doing something productive, such as cleaning up, so make sure you pay close attention to each TLC plate and exactly when each plate started running so that you know when to stop each plate.
- Using a pair of forceps, grab the top of the TLC plate and remove it from the developing chamber.
- IMMEDIATELY draw a line showing where the solvent made it on the TLC plate.
- The solvent will start evaporating immediately, so the longer you wait to draw this line, the less accurate your results will be.
- The solvent might “smile”, meaning that the solvent closer to the edges ran slightly faster than the solvent in the middle. If this happens, the line you draw should reflect this.
- Once all of the solvent has evaporated from your TLC plate (i.e., it’s no longer dark anywhere), make a sketch of the TLC plate in your lab notebook (including a ruler) and take a picture of the TLC plate (including a ruler).
Week 2: Column Chromatography
- separate the pigments using microscale column chromatography.
- measure the absorption spectrum of each of the pigments.
- silica gel (about 1-2 cm 3 )
Column Chromatography
We will now separate out the different pigments in the pigment sample you extracted above, to isolate different pigments and characterize their absorption spectra.
- Once you start the elution process, it cannot be stopped. You must go to completion. Be sure to read all directions for this part before proceeding. Everything must be staged so that the process can occur seamlessly.
- One of the most important rules is that columns must not be allowed to go dry. A continual flow of solvent is therefore necessary to ensure that the resin remains wet and intact.
- As glass cuvettes are fragile, be sure to handle these carefully. These will need to be shared between all members of the class.
- The first fraction eluted is beta-carotene, and the second is chlorophyll (you may be able to separate those further, but likely not).
- If necessary, add ~0.4 mL of hexane (measure using a transfer pipet) to your vial and gently mix to reconstitute (redissolve) your pigments.
- Get a small piece of cotton or glass wool and wedge this into the narrow end of a short Pasteur pipet the same way you did last week for the filtering pipet. This should be relatively loose so liquid can get through but solid cannot.
- Scoop silica gel carefully into the column using a weigh paper funnel and make sure the column is collapsed so the powder falls to the bottom. Fill the pipet until it is about 2/3 full.
- Gently tap the side of the pipet for one minute to pack the silica.
- Add 0.5 cm of sand to the top of the pipet.
- Place the pipet column in a pipet clamp and secure it to the ring stand.
- Prepare 2 clean, small test tubes to collect the column fractions and a 100 mL beaker for waste. Label the TOP of the test tubes with your initials, the date and what fraction you will collect in that test tube (fraction 1 or fraction 2).
- Obtain 15 mL of acetone and 15 mL of hexane in separate beakers (keep these beakers covered as much as possible to minimize evaporation).
- Before elution, the column must be equilibrated (i.e. made to have similar solvent conditions as the mobile phase). To do this, place the waste beaker under the pipet column and elute 4-6 mL of hexane through the column. Note: If air bubbles or cracks appear in the column, discard and repeat Step 2.
- Note: It is important here that while there is a little mobile phase left, there isn’t too much hexane left on top of the column. If there is too much then this would dilute the pigment solution and “blur” the separation between the pigments.
- Allow the solution to adsorb onto the silica by letting it run into the column. Once the solution has adsorbed into the column (moved just below the sand) but before the silica is exposed to air, continue adding hexane continuously. Observe and record the migration of the bands that appear. Continue collecting all clear liquids in a waste beaker.
- A yellow band will appear and begin to separate from the green band. Continue adding hexane until you finish collecting the yellow fraction in a clean test tube.
- Once you have collected the yellow fraction, change the solvent by adding acetone instead of hexane. Continue adding the solvent and collect the clear portion in the waste beaker.
- The green band should be moving down the column. Collect this fraction in a clean test tube.
Note: Not all of the pigments will be removed from the column.
- Measure the absorption spectrum of each of the fractions using a small test tube (instead of a cuvette).
Week 3/4: High Performance Liquid Chromatography (HPLC)
You will analyze the final leaf extract using HPLC. The column we use is a C-8 column with 5 mm diameter (4.6 mm internal diameter x 100 mm length). The solvents used are:
- Solvent A: 80:20 methanol:water
- Solvent B: 95:10 acetonitrile:water
These solvents are a mixture of methanol (CH 3 OH) and water or acetonitrile (CH 3 CN) and water. A uniform solvent is used for elution. We will measure the absorbance of the sample at 440 nm and use this to generate the chromatograph.
The method is as follows with a flow rate of 1 mL/min
- 0 min-5 min: linear gradient from 100% solvent A to 100% solvent B
- 5 min-20 min: 100% solvent B
Your instructor will provide more information about the method closer to the time of the experiment.
- Your instructor will do this step: Load the method directed by your instructor and allow the system to equilibrate.
- The system needs to be equilibrated so there’s nothing in the column before you start another round. The chromatogram should be blank for a couple of minutes before you start.
- Look around and make sure there are no leaks in the system.
- Clean the syringe by drawing up 50:50 hexane:acetone mixture into the syringe and ejecting it into waste.
- Make sure the manual injector is in the upward direction.
- Get the sample vial from last week and redissolve in a small amount of 50:50 hexane:acetone mixture.
- Gently draw up a little more than 20 μL of the liquid with the special syringe, making sure there are no air bubbles. If there are air bubbles, eject all the liquid and try again more slowly, making sure that the tip of the needle is always submerged in the liquid. Inject the sample into the injector.
- When the injection is completed, push the injector knob downwards so that the sample is injected. Make sure no air bubbles are injected it into the system. The HPLC system will record the starting time.
- Wait until the method is complete. Make a PDF of the report and email the PDF to yourself.
Sharing of Data
You will post the PDF along with information about the leaves (species and overall appearance) onto Canvas by midnight the day you complete your lab report.
Data Analysis
- Locate spectra of different plant pigments and compare against the spectra for the pigments you extracted in the first week. Could you identify these pigments?
- For the HPLC experiment, refer to Wright, S. W. et al, Improved HPLC method for the analysis of chlorophylls and carotenoids from marine phytoplankton . Mar. Ecol. Prog. Ser. 1991, 77 , 183-196 and compare the peaks observed to yours. Note that since the method is slightly different I would not expect your spectra to look exactly the same as the spectra in this paper.
Acknowledgment:
The extraction and column chromatography procedure is based on a protocol used previously for extracting pigments from spinach leaves for organic chemistry.
- For further details, please read Hoefnagels, Biology: The Essentials , 4th Ed, Ch. 5.2 (Photosynthetic Pigments) or OpenStax Biology 2e , Ch. 8.2 . ↵
IU East Experimental Chemistry Laboratory Manual Copyright © 2022 by Yu Kay Law is licensed under a Creative Commons Attribution-NonCommercial 4.0 International License , except where otherwise noted.
Share This Book
Academia.edu no longer supports Internet Explorer.
To browse Academia.edu and the wider internet faster and more securely, please take a few seconds to upgrade your browser .
Enter the email address you signed up with and we'll email you a reset link.
- We're Hiring!
- Help Center
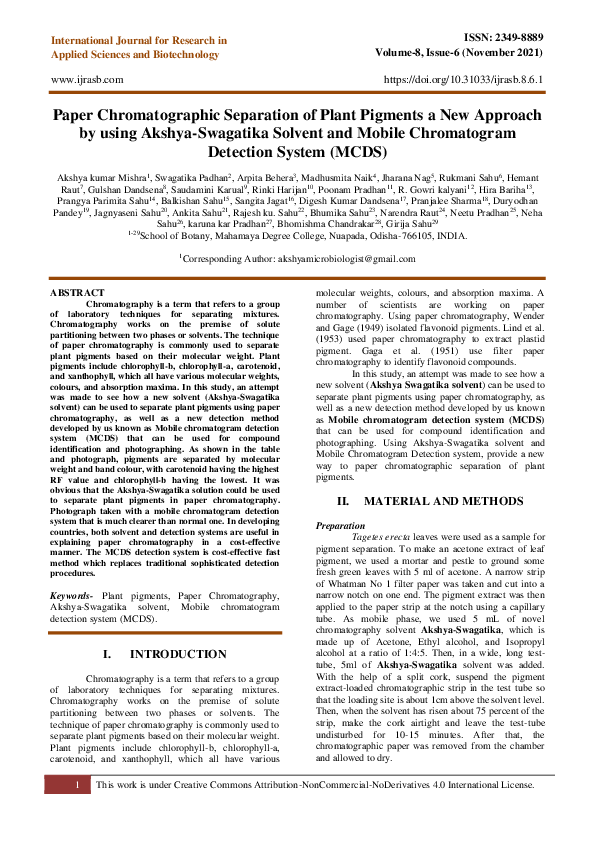
Paper Chromatographic Separation of Plant Pigments a New Approach by using Akshya-Swagatika Solvent and Mobile Chromatogram Detection System (MCDS

Related Papers
Alen Albreht
Japanese knotweed (Fallopia japonica Houtt.) and Bohemian knotweed (Fallopia x bohemica) are invasive alien plant species, causing great global ecological and economic damage. Mechanical excavation of plant material represents an effective containment method, but it is not economically and environmentally sustainable as it produces an excessive amount of waste. Thus, practical uses of these plants are actively being sought. In this study, we explored the carotenoid profiles and carotenoid content of mature (green) and senescing leaves of both knotweeds. Both plants showed similar pigment profiles. By means of high performance thin-layer chromatography with densitometry and high performance liquid chromatography coupled to photodiode array and mass spectrometric detector, 11 carotenoids (and their derivatives) and 4 chlorophylls were identified in green leaves, whereas 16 distinct carotenoids (free carotenoids and xanthophyll esters) were found in senescing leaves. Total carotenoid c...
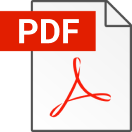
Journal of insect science (Online)
Gabby Zhang
This study used the larval tissues and colored cocoons of silkworms, Bombyx mori L. (Lepidoptera: Bombycidae), that were fed leaves of cultivated mulberry, Husang 32, as experimental material. The pigment composition and content in colored cocoons and tissues of the 5th instar larvae and the mulberry leaves were rapidly detected using organic solvent extraction and reverse phase high-performance liquid chromatography with diode array detection. It was found that the mulberry leaf mainly contained four types of pigment: lutein (30.86%), β-carotene (26.3%), chlorophyll a (24.62%), and chlorophyll b (18.21%). The silk glands, blood, and cocoon shells of six yellow-red cocoons were used as the experimental materials. The results showed that there were generally two kinds of carotenoids (lutein and β-carotene) in the silk gland and cocoon shell, a little violaxanthin was detected in silk gland, and the pigment found in the blood was mainly lutein in all varieties of silkworm tested. Chlo...
Bioresources and Bioprocessing
Mohd Azmuddin Abdullah
Background Microalgae is one of the major sources of natural compounds with antimicrobial activity. The metabolite profiling of the extracts could identify the bioactive compounds based on methanol (MET), ethanol (ETH), chloroform (CHL), hexane (HEX) and water (W) solvent systems. The microalgal crude extracts in co-application with silver nanoparticles (AgNPs) had enhanced antimicrobial activity with potential to overcome the global problem of microbial antibiotic resistance. Results Chlorella sp. exhibited the highest lipid, N. oculata the highest total saturated fatty acids (TSFA), and T. suecica the highest mono-unsaturated (MUFA) and poly-unsaturated fatty acids (PUFA). The highest carbohydrate, protein and total phenolics contents (TPCs) were attained by N. oculata. The highest total flavonoids contents (TFCs), and chlorophyll a and b were in T. suecica, while comparable level of carotenoids were found in all species. For high-performance thin-layer chromatography (HPTLC) anal...
Biodiversitas Journal of Biological Diversity
Yeni Sulistiyani
Marine Ecology-Progress …
Francisco Rodriguez
Francesca Rigano
This study aimed to investigate the potential of four sea water microalgae, isolated and cultivated at M′diq Bay in Morocco, as a new source of natural antioxidants. These microalgae belong to different classes, including Phaedactylium tricornitum (Bacillariophyceae), Nannochloropsis gaditana (Eustigmatophyceae), Nannochloris sp (Trebouxiophyceae), and Tetraselmis suecica (Chlorodendrophycea). The antioxidant properties were screened by the use of in vitro assays, namely 2,2-difenil-1-picrylhydrazyl, Ferric reducing antioxidant power, and Ferrous ions chelating activity, and compoundidentification was carried out in methanol and acetone extracts of both dried and fresh microalgae biomass by HPLC–PDA–MS analysis. Among the investigated microalgae, Phaedactylium tricornutum was the richest one regarding its carotenoid (especially all-E-fucoxanthin) and phenolic (especially protocatechuic acid) contents, as well as antioxidant activity (65.5%), followed by Nannochloris sp, Tetraselmis ...
Potravinarstvo Slovak Journal of Food Sciences
Renny Indrawati
The production of tea dregs is continually increasing along with the growth of people's interest in ready-to-drink beverages. However, the recent development of research on the use of tea dregs is still very limited. The present study was aimed to identify the remnant photosynthetic pigments in tea dregs, determine their composition, and evaluate their potential use as natural antibacterial agents based on light-induced reaction (photosensitization). The tea dregs from six commercial teas, consisting of green and black teas, were analyzed using high-performance liquid chromatography (HPLC) with a photodiode array detector, and the spectroscopic data were analyzed from 350 to 700 nm. Pigment identification was performed based on spectral characteristics, and pigment composition in the extracts from the dregs was determined by a three-dimensional multi-chromatogram analysis method. The dominant pigment fractions in both tea types were pheophytin a and its isomers, as well as pheop...
Scientia Horticulturae
Kathy Schwinn
Neelima Mahato
Citrus contains a range of highly beneficial bioactive compounds, such as polyphenols, carotenoids, and vitamins that show antimicrobial and antioxidant properties and help in building the body's immune system. On consumption or processing, approximately 50% of the fruit remains as inedible waste, which includes peels, seeds, pulp, and segment residues. This waste still consists of substantial quantities of bioactive compounds that cause environmental pollution and are harmful to the ecosystem because of their high biological oxygen demand. In recent years, citrus cultivation and the production of processed foods have become a major agricultural industry. In addition to being a substantial source of economy, it is an ideal and sustainable and renewable resource for obtaining bioactive compounds and co-products for food and pharmaceutical industries. In the present article, the various methods of extraction, conventional and modern, as well as separation and isolation of individual bioactive compounds from the extraction mixture and their determination have been reviewed. This article presents both aspects of extraction methods, i.e., on a small laboratory scale and on an industrial mass scale. These methods and techniques have been extensively and critically reviewed with anticipated future perspectives towards the maximum utilization of the citrus waste.
Journal of Agricultural and Food Chemistry
Lourdes Gallardo-Guerrero , María Roca
RELATED PAPERS
Gustaaf Hallegraeff
Canadian Journal of Botany
Constance Nozzolillo
Crestie Contrata
International Journal of Food Science & Technology
Farouk El-Baz
Journal of Chromatography A
Anita Oberholster
Food Chemistry
Maria-paz Romero
Separations
Tereza Fábryová
Joanna Molga
Journal of the Brazilian Chemical Society
Karina Cardozo
David Janero
Rohmah Fitriyani
Kevser Sözgen Başkan
Analytical and bioanalytical …
Mladen Franko , Alenka Malej
Yasser Abdelhady , Salah Saleh
Francisco Delgado-Vargas
Rapid Communications in Mass Spectrometry
Antonio Coletta
MARIA CLAUDIA DUSSI
Hussein Daood
Dominic Hodgson
Martine Bertrand
Food Science and Applied Biotechnology
Petar Zhelev
Jennifer Elliott
F. Torregrosa , Ana Frígola
New Phytologist
Sofia Moco , Arjen Lommen
Marine Ecology Progress Series
Bente Edvardsen
sailesh tas
Procedia Chemistry
Leenawaty Limantara
Journal of Chromatographic Science
Emilio Monge Pacheco
George Radu
Vandana Publications
International Journal for Research in Applied Sciences and Biotechnology Journal , Mayank Chauhan
Dr shivayogeeswar Neelagund
Dominic Petrella , Ed Nangle , James Metzger
Food Research International
Leila Queiroz Zepka
International Journal of Analytical Chemistry
Gaurav Rajauria
Photosynthesis research
Progress in Oceanography
Ben Wigham , Patricia Pérez-barros
Pharmaceutical …
Dr. Bakrudeen Ali Ahmed Abdul
- We're Hiring!
- Help Center
- Find new research papers in:
- Health Sciences
- Earth Sciences
- Cognitive Science
- Mathematics
- Computer Science
- Academia ©2024

Separation of Plant Pigments by Paper Chromatography
The separation of plant pigments by paper chromatography is an analysis of pigment molecules of the given plant. Chromatography refers to colour writing . This method separates molecules based on size, density and absorption capacity.
Chromatography depends upon absorption and capillarity . The absorbent paper holds the substance by absorption. Capillarity pulls the substance up the absorbent medium at different rates.
Separated pigments show up as coloured streaks . In paper chromatography, the coloured bands separate on the absorbent paper. Chlorophylls, anthocyanins, carotenoids, and betalains are the four plant pigments.
This post discusses the steps of separating plant pigments through paper chromatography. Also, you will get to know the observation table and the calculation of the Rf value.
Content: Separation of Plant Pigments by Paper Chromatography
Paper chromatography, plant pigments, steps of plant pigment separation, observation, calculation.
It is the simplest chromatography method given by Christian Friedrich Schonbein in 1865. Paper chromatography uses filter paper with uniform porosity and high resolution.
The mixtures in compounds have different solubilities . For this reason, they get separated distinctly between the stationary and running phase.
- The mobile phase is a combination of non-polar organic solvents. The solvent runs up the stationary phase via capillary movement.
- The stationary phase is polar inorganic solvent, i.e. water. Here, the absorbent paper supports the stationary phase, i.e. water.
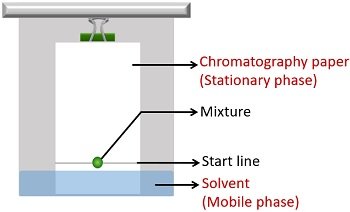
Plant pigments are coloured organic substances derived from plants. Pigments absorb visible radiation between 380 nm (violet) and 760 nm (red).
They give colour to stems, leaves, flowers, and fruits. Also, they regulate processes like photosynthesis, growth, and development.
Plants produce various forms of pigments. Based on origin, function and water solubility, plant pigments are grouped into:
- Chlorophylls (green)
- Carotenoids (yellow, orange-red)
- Anthocyanins (red to blue, depending on pH)
- Betalains (red or yellow)
Chlorophyll : It is a green photosynthetic pigment. Chlorophyll a and b are present within the chloroplasts of plants. Because of the phytol side chain, they are water-repelling . Their structure resembles haemoglobin. But, they contain magnesium as a central metal instead of iron.
Carotenoids : These are yellow to yellow-orange coloured pigments. Also, they are very long water-repelling pigments. Carotenoids are present within the plastids or chromoplasts of plants.
Anthocyanins : These appear as red coloured pigments in vacuoles of plant cells. Anthocyanins are water-soluble pigments. They give pink-red colour to the petals, fruits and leaves.
Betalains : These are tyrosine derived water-soluble pigments in plants. Betacyanins (red-violet) and betaxanthins (yellow-orange) are the two pigments coming in this category. They are present in vacuoles of plant cells.
You can separate all the above pigments using paper chromatography.
Video: Separation of Plant Pigments
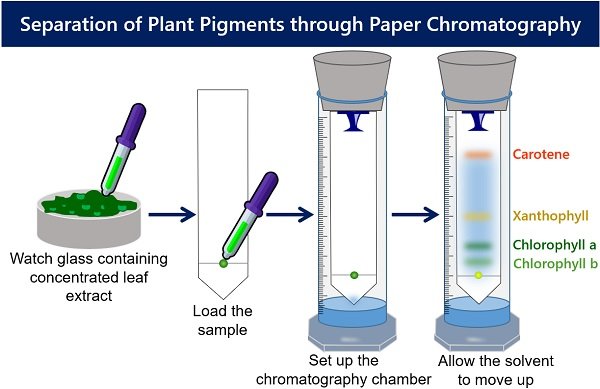
Preparation of Concentrated Leaf Extract
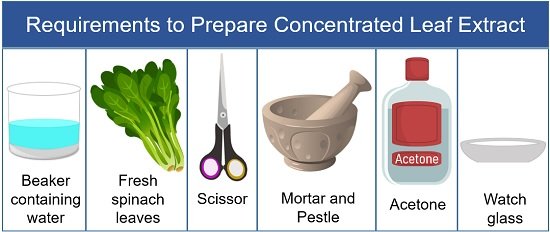
- Wash spinach leaves in distilled water.
- Then take out the spinach leaves and allow the moisture to dry out.
- After that, take a scissor and cut the leaves into the mortar.
- Take a little volume of acetone into the mortar. Note : Acetone is used instead of water to mash the leaves because it is less polar than the water. This allows a high resolution of the molecules in the sample between the absorbent paper.
- Then, grind spinach leaves using a pestle until liquid paste forms. Note : The liquid in the crushed leaf paste is the pigment extract.
- After that, take out the mixture into the watch glass or Petri dish.
Load the Leaf Extract onto Absorbent Paper
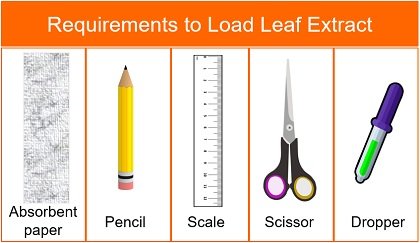
- Take Whatman filter paper and draw a line above 2 cm from the bottom margin. You can use a pencil and scale to draw a fainted line. Note : A pencil is used because pencil marks are insoluble in the solvent.
- Then, cut the filter paper to make a conical edge from the line drawn towards the margin end. You can use a scissor to cut the Whatman filter paper. Note : The conical end at the bottom of the filter paper results in better separation.
- Put a drop of leaf extract on the centre of a line drawn on the absorbent paper.
- Then, at the same time dry the absorbent paper.
- Repeat the above two steps many times so that the spot becomes concentrated enough.
Setup the Chromatography Chamber
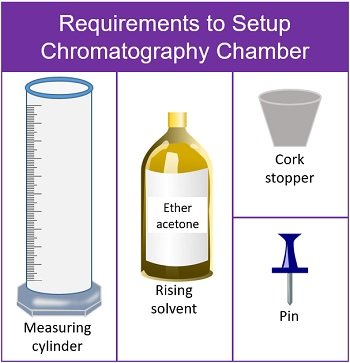
- Take a clean measuring cylinder and add rising solvent (ether acetone) up to 4 ml.
- Bend the strip of paper from the top. Then, using a pushpin attach the paper to the bottom of the cork.
- Adjust the length of the paper. The absorbent paper should not touch the surface of the measuring cylinder.
- After that, allow the solvent to move up the absorbent paper.
- When the solvent front has stopped moving, remove the paper.
- Allow it to dry for a while until the colours completely elute from the paper.
- At last, mark the front edge travelled by each pigment.
Over the dried paper strip, you will see four different bands. Different colour streaks form because of different affinities with the mobile phase (solvent).
- The carotene pigment appears at the top as a yellow-orange band.
- A yellowish band appears below the carotene, which indicates xanthophyll pigment.
- Then a dark green band represents the chlorophyll-a pigment.
- The chlorophyll-b pigment appears at the bottom as a light green band.
Observation Table
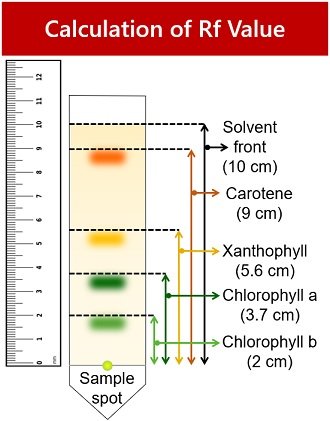
1. Light green spot indicates chlorophyll-b pigment.
- Rf value= Distance chlorophyll-b travelled / Distance solvent travelled = 2/10 = 0.2
2. Dark green spot represents chlorophyll-a pigment.
- Rf value= Distance chlorophyll-a travelled / Distance solvent travelled = 3.7/10 = 0.37
3. The yellow band represents xanthophyll pigment.
- Rf value= Distance xanthophyll travelled / Distance solvent travelled = 5.6/10 = 0.56
4. The yellow-orange band indicates carotene pigment.
- Rf value= Distance carotene travelled / Distance solvent travelled = 9/10 = 0.9
Factors affecting the Rf values of a particular analyte are:
- Stationary phase
- The concentration of the stationary phase
- Mobile phase
- The concentration of the mobile phase
- Temperature
The Rf value of compounds in the mixture differs by any changes in the concentration of stationary and mobile phases.
Temperature affects the solvent capillary movement and the analyte’s solubility in the solvent. Rf value is independent of the sample concentration. Its value is always positive .
Related Topics:
- Difference Between Budding and Grafting
- Phototropism in Plants
- Potometer Experiment
1 thought on “Separation of Plant Pigments by Paper Chromatography”
Nice experiment and understanding.
Leave a Comment Cancel Reply
Your email address will not be published. Required fields are marked *
Start typing and press enter to search
- Biology Article
- Separation Of Plant Pigments Through Paper Chromatography
How to Separate Plant Pigments Using Paper Chromatography
To distinguish and study the various pigments present in plants through the process of paper chromatography.
Plants carry out the process of photosynthesis, during which light energy from the sun is converted into chemical energy (food). The capturing of light energy is carried out by molecules known as pigments, which are present within the plant cells.
Explore more: Plant cells .
What are Pigments?
Pigments are chemical compounds, which are able to reflect only a particular range of wavelengths of visible light. Leaves of plants primarily contain different types of pigments within their tissues. The four different types of pigments are listed below in a tabular column along with their colours.
In order to view and distinguish the primary four plant pigments, a simple technique known as chromatography can be used.
Read more: Pigments
What is Chromatography?
It is a technique that is used to distinguish between different molecules. This differentiation is based on these attributes-shape, size, charge, mass, adsorption and solubility.
Types of chromatography:
- Column chromatography
- Paper chromatography
- Partition chromatography
- Thin-layer chromatography
Mechanism of Paper Chromatography
In this technique, the interaction between three components is involved – solid phase, separation of a mixture and a solvent.
- At first, the mixture is spotted onto the paper and is dried.
- The solvent is made to flow through the capillary attraction.
- While the solvent moves through the paper, the various components of the mixture differentiate into varied coloured spots.
- Later the paper is allowed to dry and the position of various compounds is viewed.
- The substance, which is the most soluble moves further on the paper as compared to the other substances that are less soluble.
Material Required
- Chromatography chamber
- Spinach leaves
- Mortar and pestle
- Ether acetone solvent
- Capillary tube
- Filter paper strips
- Watch glass
- In this experiment, spinach leaves are used to separate different pigments.
- Pick a few fresh and green leaves of spinach and wash it.
- Cut out small pieces of spinach using scissors. Add them to the mortar.
- Accurately measure 5ml acetone using a measuring cylinder and add it into the mortar.
- With the help of mortar and pestle, grind the spinach leaves into a smooth paste.
- Shift the prepared paste of spinach into the watch glass with the help of a spatula.
- Place a filter paper strip with a tapering notch towards one ending of the strip.
- Horizontally trace a line with a scale and a pencil that is 2 to 3 cm apart from the notch’s tip.
- Using a capillary tube, add 1 drop of the extract of the pigment in the midsection of the line.
- Let the drop dry. Repeat the same process of adding a drop and allowing it to dry for 4-5 times.
- In the chromatographic chamber, pour the ether acetone solvent.
- Make sure to folded and stapled an end side of the paper.
- Suspend the strip in the chamber.
- The loading spot remains about 1 cm above the level of the solvent.
- Let the chamber remain uninterrupted for a while.
- We can notice that the solvent passes along the paper scattering various pigments of the blend to different distances.
- Once the solvent reaches 3/4 th of the strip, carefully take the strip off.
- Allow the strip to dry.
Observation
The dried paper strip displays four different bands. Discrete pigments can be distinguished with the help of colours.
- The Carotene pigment is observed at the topmost as an orange-yellow band of pigments distinctively.
- Just below this band, a yellowish band appears which indicates the pigment xanthophyll.
- The third band appearing dark green indicates chlorophyll-a pigment.
- The yellowish-green band present at the bottom is the chlorophyll b pigment.
Precautions
- The leaves that are selected should be green and fresh spinach leaves
- From the tip of the notch, the loading spot needs to be 2 to 3 cm apart
- While suspending the filter paper strips in the chamber, one need to ensure that the loading spot needs to be set up above 1 cm from the level of the solvent.
Viva Questions
Q.1. What Rf value or Retention factor?
A.1. The Retention factor or Rf value applies to chromatography to make the technique scientific. It is defined as the distance travelled by the compound divided by the distance travelled by the solvent.
Rf value = Distance travelled by the compound / Distance travelled by the solvent.
Q.2. What is Phycobilin?
A.2. Phycobilins are light-capturing bilins found in chloroplast organelles, cyanobacteria and in a few algae.
Q.3. What is the significance of pigment in photosynthesis?
A.3. It helps in the absorption of energy from light. The free electrons in the pigments present in their chemical structure transfer their energy to other molecules during photosynthesis when they turn into high energy electrons, thereby liberating energy they captured from light. This released energy is then used up by other molecules for the formation of sugars and related nutrients with the use of water and carbon dioxide.
For more Biological concepts, experiments and other related topics, visit us at Byju’s Biology
Related Links:
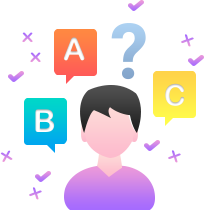
Put your understanding of this concept to test by answering a few MCQs. Click ‘Start Quiz’ to begin!
Select the correct answer and click on the “Finish” button Check your score and answers at the end of the quiz
Visit BYJU’S for all Biology related queries and study materials
Your result is as below
Request OTP on Voice Call
Leave a Comment Cancel reply
Your Mobile number and Email id will not be published. Required fields are marked *
Post My Comment

- Share Share
Register with BYJU'S & Download Free PDFs
Register with byju's & watch live videos.

- Press Enter to activate screen reader mode.
Institute of Molecular Plant Biology
Our paper made it into the eth news.
Twisted pollen tubes induce infertility Plants with multiple sets of chromosomes have advantages over their relatives with a double set. But why they often start out infertile was only partially understood. Biologists at ETH Zurich have now discovered a new reason for the initial difficulties.
- mode_comment Number of comments
Please follow link to the ETH News site for the whole article: LINK
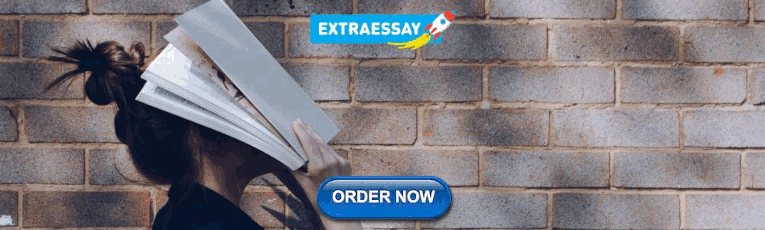
IMAGES
VIDEO
COMMENTS
The aim of this paper is to present a comprehensive overview of the main aims and scopes in screening of botanicals, a task of which thin-layer chromatography (TLC) is, on an everyday basis, confronted with and engaged in. Stunning omnipresence of this modest analytical technique (both in its standard format (TLC) and the high-performance one (HPTLC), either hyphenated or not) for many ...
2.1 Part A: Absorption Spectrum of Plant Extract. The purpose of the first part of the experiment is to observe the absorption spectrum of the. separated photosynthetic pigments; simply put, how ...
to handle paper as little as possible. 1. Cut a piece of Whatman #1 filter paper or chromatography paper to the dimensions of 12 cm X 14 cm. Edges must be straight. 2. With a pencil lightly make a line 1.5 - 2 cm from the bottom edge of the paper which measures 14 cm. 3. Select 2 large dark green spinach leaves and blot dry with paper towels.
The use of column chromatography and paper thin-layer techniques has allowed for the isolation and purification of several bioactive materials due to their availability in a variety of fixed ...
In addition, thin-layer chromatography (TLC), high-performance liquid chromatography (HPLC), paper chromatography (PC), and gas chromatography (GC) were used in separation and purification of the secondary metabolites.[1,2,3,4,5] The choice of an appropriate extraction method depends on the nature of the plant material, solvent used, pH of the ...
Column chromatography of photosynthetic plant pigments is a common experiment in introductory laboratory courses for science majors and nonscience majors alike. We report a simple, rapid, reproducible, and small-scale solid-liquid extraction of photosynthetic pigments from inexpensive, store-bought dried herbs that affords a dark-green extract solution, all of which can be applied directly ...
Background Fumaria parviflora (Lam.), commonly known as "fine-leaved fumitory," is well known for its therapeutic properties in the Indian traditional medicinal system. The presence of important bioactive compounds in plants makes them pharmacologically valuable. Therefore, in the present study, the high-performance thin layer chromatography (HPTLC) analysis of Fumaria parviflora (whole ...
Paper chromatography is a technique used to separate and identify plant pigments. • Plant pigments are not equally soluble within the chromatography solvent. As a result, they will stop at different places as the solvent wicks up the chromatography paper. • Plant pigments are colored molecules that absorb light at specific wavelengths.
Activity 2: Paper Chromatography of Plant Pigments. Paper chromatography is an analytical method that separates compounds based on their solubility in a solvent. The solvent is used to separate a mixture of molecules that have been applied to filter paper. The paper, made of cellulose, represents the stationary or immobile phase. The separation ...
Abstract. This paper summarizes the role of planar chromatography (PC) in medicinal and aromatic plant (MAP) research and development, and demonstrates the importance of the technique, after extraction, in the analysis of MAP (identification and quantitative determination of the separated compound/s), in the purification and isolation process, and in different types of screening procedure.
The aim of this paper is to present a comprehensive overview of the main aims and scopes in screening of botanicals, a task of which thin-layer chromatography (TLC) is, on an everyday basis, confronted with and engaged in. Stunning omnipresence of this modest analytical technique (both in its standard format (TLC) and the high-performance one (HPTLC), either hyphenated or not) for many ...
To improve separation, reverse-phase ion pairing chromatography, hydrophilic interaction chromatography (HILIC) and other chromatographic methods can be used; another option is chemical ...
A common screen for plant antimicrobial compounds consists of separating plant extracts by paper or thin-layer chromatography (PC or TLC), exposing the chromatograms to microbial suspensions (e.g. fungi or bacteria in broth or agar), allowing time for the microbes to grow in a humid environment, and visualizing zones with no microbial growth.
Plant Traveling Lab. TTU/HHMI at CISER. 2010 3 Preparation of the Sample: (Important! Oil from the skin affects the separation, so handle paper as little as possible and only by the edges.) 1. Take a strip of chromatography paper approximately 18 cm long. One end is blunt and the other is pointed. 2.
The Altmetric Attention Score is a quantitative measure of the attention that a research article has received online. Clicking on the donut icon will load a page at altmetric.com with additional details about the score and the social media presence for the given article. ... Normal- and Reverse-Phase Paper Chromatography of Leaf Extracts of ...
Classification [7] We can classify and summarize the chromatographic method technique into three. different ways as the following: 1) Depend on the shape of the stationary phase. e.g.- planar and ...
The objective of this paper is to provide a review of phytochemical studies that have addressed extracting, measuring and identifying bioactive compounds of plants. ... Developed instruments such as High Pressure Liquid Chromatography (HPLC) accelerate the process of purification of the bioactive molecule. ... Extensive research has been ...
Record the species and mass to the nearest 0.001 g, as well as a description of the leaves. Tear or cut the leaves into small pieces and place them in a mortar with a pinch of sand (to assist with grinding up the leaves). Add 6 mL of acetone and 6 mL of hexane and grind the mixture for 3-5 minutes in the fume hood.
Academia.edu is a platform for academics to share research papers. Paper Chromatographic Separation of Plant Pigments a New Approach by using Akshya-Swagatika Solvent and Mobile Chromatogram Detection System (MCDS ... HC Lane,LS Gleason (1953): partial separation of the plastid pigment by paper chromatography; Plant physiology 28(2),325-328 ...
1 Comment / Botany / By Supriya N. The separation of plant pigments by paper chromatography is an analysis of pigment molecules of the given plant. Chromatography refers to colour writing. This method separates molecules based on size, density and absorption capacity. Chromatography depends upon absorption and capillarity.
Using a capillary tube, add 1 drop of the extract of the pigment in the midsection of the line. Let the drop dry. Repeat the same process of adding a drop and allowing it to dry for 4-5 times. In the chromatographic chamber, pour the ether acetone solvent. Make sure to folded and stapled an end side of the paper.
Tagetes erecta Linn. is an ornamental plant containing valuable therapeutic phytochemicals. In the current investigation, ethanol extraction of the dried flower sample was subjected to column ...
Our paper made it into the ETH News. Twisted pollen tubes induce infertility Plants with multiple sets of chromosomes have advantages over their relatives with a double set. But why they often start out infertile was only partially understood. Biologists at ETH Zurich have now discovered a new ...